All published articles of this journal are available on ScienceDirect.
Synthesis of Molecularly Imprinted Polymers for the Selective Extraction /Removal of 2,4,6-trichlorophenol
Abstract
Background:
2,4,6-Trichlorophenol (2,4,6-TCP) is one of the most significant pollutants among chlorophenols due to its harmful effects. It has been classified as priority pollutants by the U.S. Environmental Protection Agency. Therefore, highly selective separation and sensitive recognition of 2,4,6-TCP from complex samples are in great demand.
Methods:
For this purpose , the preparation of MIPs selective for 2,4,6-TCP was carried out by precipitation polymerization. A non-covalent approach was employed to establish an interaction between template and monomer (methacrylic acid).
Results:
The resulted polymers were characterized by scanning electron microscopy (SEM), EDX, Fourier-transform infrared spectroscopy(FT-IR) and BET. The batch binding assay was carried out to select the most selective polymer in terms of binding efficiency towards the target template. The adsorption parameters such as initial concentration, dosage of polymer, pH effect and selectivity with structural analogues were determined . The selectivity of MIP towards the 2, 4, 6-TCP was higher as compared to its structural analogue melamine with a good adsorption efficiency. Furthermore, the MIP as an extracting material was applied for extraction of 2, 4, 6-trichlorophenol from the spiked blood serum (88%) and river water sample (94%). The results showed that the optimized MIP could successfully extract 2,4,6-TCP from the blood serum and river water.
Conclusion:
The molecularly imprinted polymers for 2,4,6-TCP have been prepared by precipitation polymerization with a non-covalent approach. The optimized MIP has been successfully used for the extraction of 2,4,6-TCP from blood serum and river water.
1. INTRODUCTION
Chlorophenols (CPs) are considered as uncontrolled environmental pollutants due to their toxicity, carcinogenicity, and bioaccumulation capability [1]. They have been extensively used in various industries and are disposed off in the form of various industrial processes [2]. Among CPs, 2,4,6-Trichlorophenol (2,4,6-TCP) is considered as one of the toxic pollutants because of its harmful effects [3-5]. The U.S. Environmental Protection Agency has also classified it as a priority pollutant [6].Chlorophenols are poisonous and possibly cancer-causing and can influence the smell and taste of drinking water even in very low concentrations (µgL-1) , therefore, it is included by both the US Environmental Protection Agency (EPA), 1977 and the European Union (EU) in their lists of priority pollutants. EU Directive 2455/2001/EC sets the most extreme 0.5 µgL-1 concentration in drinking water and individual CPs concentration must not surpass 0.1 µgL-1. This enrollment is due to the way that these compounds are found to be lethal and have extremely short and long-term impacts on humans and other living organisms [7]. Therefore, a highly selective method for the extraction and recognition of 2,4,6-TCP from complex samples is in great demand. Adsorption is the best technique amongst the analytical methods used for the determination and extraction of chlorophenols from various samples as postulated by various researchers in their studies [8-11].
Molecularly imprinted polymers (MIPs) can be easily prepared with selectivity and specificity for a target molecule. This can be achieved by simply synthesizing a highly cross-linked polymer in the presence of a template molecule. The cavities complementary in size, shape, and chemical functionality to the template are retained as memory in the polymer matrix after the removal of the template molecule [12, 13]. MIPs have many advantages, such as stable to a wide range of pressures, organic solvents, and varying pHs. MIPs have many attractive applications such as in chromatographic separations [14, 15], for molecular and ionic separations [16, 17], in sensors [18, 19], in immunoassays [20, 21], and as catalysts [22, 23]. There are various methods that have been adopted such as bulk polymerization [19], precipitation polymerization [24], emulsion polymerization [25] and suspension polymerization [26, 27] for the synthesis of MIPs. Among all the methods reported above, precipitation polymerization is very convenient to produce polymer particles of uniform shape and size. Also, there is a need for a fast and straight forward method for the preparation of spherical imprinted polymers with high selectivity . Therefore, MIPs selective for 2,4,6-TCP were prepared by precipitation polymerization. In this study, a non-covalent approach was adopted to establish an interaction between a template and a monomer. Methacrylic acid was used as a monomer to interact with the 2,4,6-TCP.
2. MATERIALS AND METHODS
2.1. Materials
2,4,6-trichlorophenol (2,4,6 TCP), Divinylbenzene (DVB), 2, 2-azobisisobutyronitrile (AIBN), hydrochloric acid(HCl) and sodium hydroxide(NaOH) were purchased from Sigma-Aldrich Co. Ltd. (United States). Methacrylic acid (MAA) was bought from Nippon Shokubai Co.Ltd.(Japan). Acetonitrile (ACN), methanol (MeOH) and acetic acid (CH3COOH) were obtained from R& M chemicals. Potassium bromide (KBr) was obtained from Powder Pack Chem Co. (India).
2.2. Equipment
An ultrasonic cleaner (Branson 2510), Memmert W350T Water Bath AAR 3060, Thermo ScientificNicoletiS10 (FTIR), Scanning electron microscope (JEOL JSM-6390LA), Shaker (NB-101MT), Centrifuge (EBA20-Hettich) and, reversed-phase high-performance liquid chromatography (RP-HPLC) (ShimadzuLC-20A) were used.
2.3. Preparation of Molecular Imprinted Polymers
The synthesis method was adopted from the previous studies with a high degree of modification which includes the type of solvent, monomers and crosslinker used, the temperature range and duration of polymerization [28-30]. The synthesis of the imprinted polymer was carried out by dissolving first 2, 4, 6-TCP (1 mmol), in 80 mL of acetonitrile (ACN) followed by the addition of the functional monomer MAA, cross-linker DVB and an initiator AIBN. The solution was sonicated for 15 min and then deoxygenated with nitrogen for 15 min. After that, the reaction flask was sealed and placed in a 60˚C water bath and polymerized for 4 hours and then the temperature was increased and kept constant at 80oC for 2 hours in order to complete the polymerization process. The polymer particles obtained were centrifuged for 20 min at 6000 rpm. After removing the supernatant, the polymers were washed with the acetone. Finally, the particles were dried in an oven for six hours at 60˚C. The same procedure was followed for the preparation of other MIPs and NIP as listed in Table 1. The NIP was prepared in the absence of a template molecule. All the optimized conditions and concentrations of the template, monomer, and cross-linker were selected from the results of preliminary studies.
2.4. Removal of 2, 4, 6-TCP from Molecularly Imprinted Polymers (MIPs)
The template molecule was completely extracted from the synthesized MIPs by washing with the mixture of methanol/acetic acid (90:10, v/v). The removal of the template was monitored by HPLC at 280 nm. After the removal of the template, the polymer particles were washed with acetone to remove residual acids from the MIPs. The particles were then dried in an oven for 6 hours and weighted.
2.5. Rebinding Assay
In this experiment, 10 mL of 30 ppm 2,4,6-TCP was added into different flasks containing 100 mg washed and dried MIPs (MIP 1, MIP2, MIP3) respectively. The flasks containing the solution were agitated on a shaker at room temperature for 6 hours. The flasks were collected at the time interval of 30 min (30, 60, 90, 120, 150, 180, 210, 240, 270, 300, 330 and 360 min). The concentration of free 2,4,6-TCP was monitored using HPLC. The RP-HPLC was conducted by using methanol, acetonitrile and ultra-pure water (70:20:10, v/v/v) as an eluent and C18 column as a stationary phase. The flow rate of the sample was maintained at 0.6 mL/min with an injection volume of 20 µl. The rebinding efficiency was calculated by using the following equation.
![]() |
(1) |
Where Ci and Cf are the initial and final concentrations of 2,4,6-TCP in the feed solution, respectively.
2.6. Adsorption Studies
The parameters affecting binding efficiency such as contact time, initial 2,4,6-TCP concentration, polymer dosage and pH value on the adsorption of 2,4,6-TCP were studied by batch binding experiments. All the parameters were selected on the basis of preliminary studies as shown in Table 2. The same procedure was followed in all the cases as in rebinding assay.
The selectivity of the MIP and NIP towards 2,4,6-TCP was compared with its structural analogue compound, melamine. Both the templates with an equal volume (5 mL) and concentration (15.0 ppm) were mixed together and were treated with MIP and NIP (200 mg) in two different flasks. After that, both the flasks were kept on a shaker for 90 min at 250 rpm. The concentrations of melamine and 2,4,6-TCP were determined by RP-HPLC. The extraction efficiency of MIP and NIP was calculated by using the following equation,
![]() |
(2) |
Where kd is the distribution coefficient, Ci and Cf are the initial and final concentration of the solutions, V and M are the volume of the solution and mass of MIP or NIP used, respectively.
The selectivity coefficient (kTCP-MEL) was calculated by using Eq. 3 as follows:
![]() |
(3) |
Where Kd TCP and Kd MEL are the distribution coefficient of 2,4,6-TCP and melamine, respectively.
The relative selectivity coefficient, K′ can be calculated by using Eq. 4.
![]() |
(4) |
Where, kMIP and kNIP are the distribution coefficients of imprinted and non-imprinted polymers, respectively [21].
3. RESULTS AND DISCUSSION
3.1. Synthesis of Molecularly Imprinted Polymers
The 2, 4, 6-TCP-MIPs were successfully synthesized by copolymerization of methacrylic acid (MAA) as shown in Fig. 1. In 2, 4, 6-TCP-MIP synthesis, H-bonding and halogen bonding were the dominant non-covalent interactions between the functional monomer MAA and the template (2, 4, 6-TCP). The degree of bonding and molar ratio of the monomer used were determined by the number of active binding sites on 2, 4, 6-TCP molecule. The carboxyl group of MAA facilitated H-bonding between the C-H groups of templates. A numbers of halogen bonds were found to be operative between the Cl group of template and hydrogen groups of functional monomers as shown in Fig. (1).
3.2. Chemical Characterization
FTIR spectroscopy is one of the most important and valuable techniques used to determine the functional groups present within the molecule. The FTIR spectra of MIPs and NIP are shown in Fig. (2). The absorption band at 3436-3466 cm-1 of the MIPs is assigned to the stretching vibration of O-H bonds from MAA molecules. The peaks around 1,722, 1,257 and 1,165cm−1 of MIPs and NIP were attributed due to C=O stretching vibration of carboxyl (MAA), C-O symmetric and asymmetric stretching vibrations, respectively [31]. The two bands 2988 and 2951 cm-1 observed were attributed due to the symmetric and antisymmetric stretching vibrations of the methylene group [22, 23]. The stretching vibrations C=C were observed with a very strong band at 1638-1640 cm−1. The C–H bending bands and C–H out of the plane, in CH2 gave medium vibration bands in the range of 1454-1469 cm−1 and 835–847 cm−1, respectively. The O-H bending and deformation vibrations were observed at 1388 and 1257 cm-1, respectively. The characteristic peak at 656 cm-1 was assigned as a C-Cl bond stretching vibration [32]. The peaks at 2988 and 2996 cm- 1, indicated the presence of C-H stretching bands. All the results confirmed the initiation of copolymerization of MAA and DVB in the presence of AIBN .
3.3. Scanning Electron Microscope (SEM)
The surface morphology (SEM images) of both MIP and NIP is shown in Fig. (3a & 3b) . Both the MIP and NIP particles produced were uniform in shape and size. This might be because of the precipitation polymerization method used for the synthesis of polymers. It has been reported that the precipitation polymerization method is a very flexible method for the production of microspheres with a uniform shape and size [28-30]. Another reason could be the use of acetonitrile as a solvent for the synthesis of polymers. This has been reported that in the acetonitrile medium, a uniform shape and size with good porosity of polymers were observed . Another factor could be the presence of divinylbenzene as a cross-linker in the polymer matrix [28-31, 33]. The size of NIP particles is almost double to that of the MIP particles, which suggests that the template molecule greatly influences the growth of imprinted particles during the process of polymerization. The functional monomers exist in two forms in the pre-polymerization mixture, such as in free form and in the dimer form. In the absence of a proper template molecule, the monomers (MAA) form H-bonded dimers in the non-imprinted pre-polymerization mixture. It is the molecular interaction between the template and monomer molecules which affects the growth of MIP particles during polymerization. In imprinted polymers, the template molecule acts as a nucleon and attracts the monomers towards it, shrinking the size of MIP particles [29] as proved from the SEM images of MIP and NIP in Fig. (3a & 3b).
3.4. EDX Analysis
The amount of main chemical constituent present in the polymer backbone such as carbon (C) and oxygen (O) was quantitatively estimated by energy-dispersive X-ray (EDS) analysis. The EDX analysis was recorded for unwashed MIPs from which the template molecules were not leached to determine the incorporation of template molecules within the polymer chain. It was (Fig. 4) observed that a significant amount of carbon (61.75%) and oxygen (31.64%) was present in the polymer. A small amount of chlorine (6.61%) was also present in the imprinted polymer. The presence of chlorine indicates the presence of 2,4,6-TCP in the polymer. The greater percentage of carbon content shows that most of the polymer chain is made up of carbon molecules that come from the functional monomer MAA, the cross-linker DVB and also from the template 2, 4, 6-TCP. The presence of oxygen within the MIP is due to template 2, 4, 6-TCP and the functional monomer MAA, while the presence of chlorine is because of the template molecule only.
3.5. BET of MIP and NIP
The specific surface area, pores diameter, and pores volume of MIP and NIP are reported in Table 3. The results observed that the specific surface area, an average diameter of pores and volume of pores of MIP were greater than that of NIP. This indicated that a cavity created during the synthesis of MIP in the presence of a template. The presence of the template effectively restricted the decrease in the pore size in the polymerization process. Therefore, the synthesized MIP had a greater pore size and pore volume than NIP. This also indicated the formation of a compatible dimensional structure in the MIP for the selective binding of the template. BET analysis also provided information about the existence of the template in the synthesis process, suggesting that it could be the possible way for the selective removal of templates from different samples. BET analysis was conducted for MIPs after being washed to determine the porous cavities within the MIP left by the template molecule. The BET results in Table 3 depict the porous nature of MIPs and this porous nature of MIP particles is attributed to the porogen ACN used as a solvent during polymerization. According to previous research, ACN is a good porogenic solvent and has a high degree of porosity to the imprinted polymers [33].
3.6. Batch Binding Assay
The batch rebinding assay was used to evaluate the efficiency of rebinding of a template by the MIPs. In this study, three different compositions of MIPs were tested. From Figs. (5 and 6), it is clear that the MIP3 has achieved higher rebinding efficiency as compared to MIP1, MIP2 and NIP. This may be due to the formation of a good number of complementary binding sites within the polymer for the template molecule during synthesis. This indicated that the molar composition of MIP3 (1:5:10, T:M: CL, respectively) is most suitable for the preparation of imprinted polymer for 2,4,6-TCP. The rebinding efficiency in case of MIP1 and MIP2 was lower as compared to MIP3. This may be because of the presence of less binding sites within the polymer matrix. MIP3 was selected for all other studies, including adsorption study, different initial concentration, polymer dosage, pH study and effect of contact time because of its highest binding efficiency.
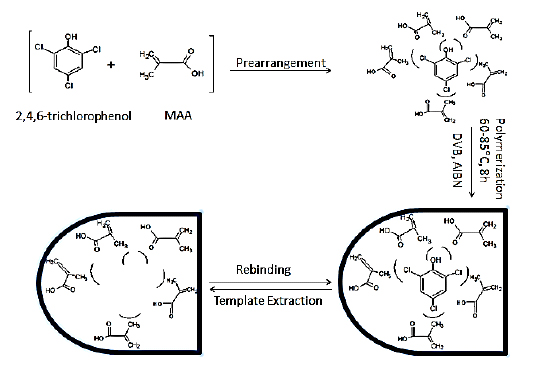
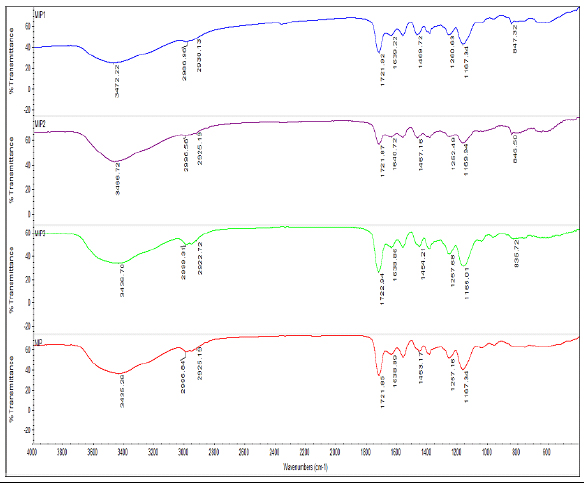
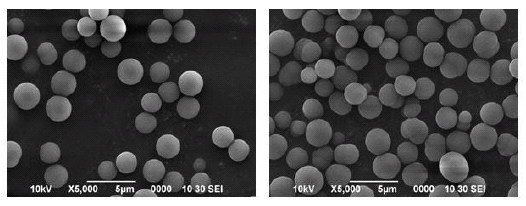
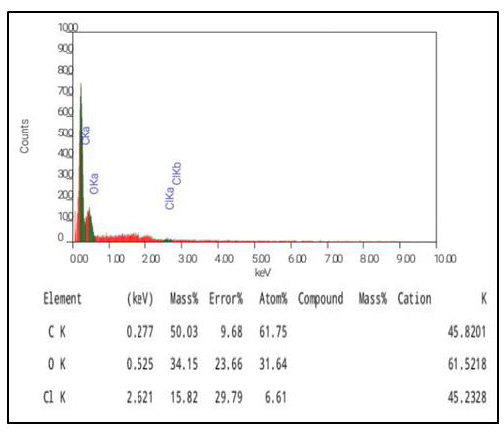
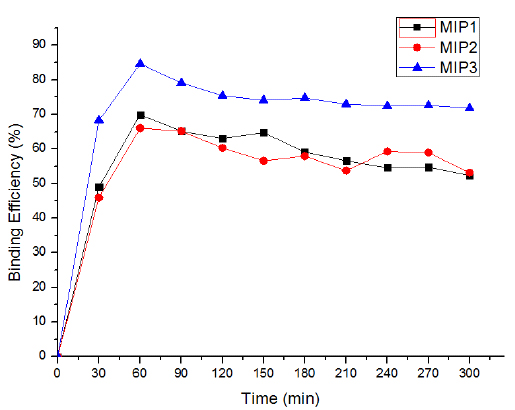
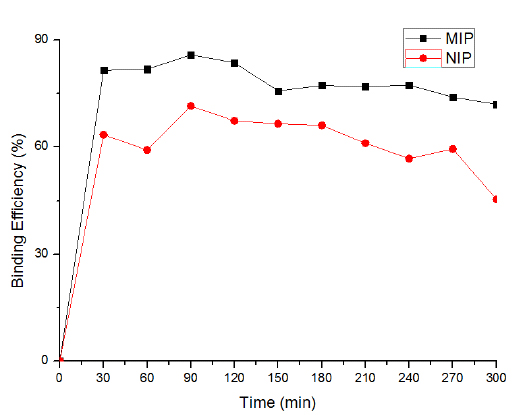
3.7. Effect of Contact Time
The effect of contact time between adsorbate and adsorbent is considered as one of the essential parameters that affect the performance of adsorption processes [34].To explain the adsorption process successfully and apply adsorbents for non-theoretical use, contact time plays a crucial role. In this study, both MIP and NIP were used to observe the effect of contact time and their comparative rebinding efficiency. It is clear from Fig. (7) that with the increase in contact time up to 90 min, the rebinding efficiency (85.78%) also enhanced. Further increase in contact time slightly decreased the rebinding efficiency which may be because of the saturation of binding sites with the template molecule. The comparative rebinding efficiency of MIP and NIP indicated that the MIP had higher rebinding efficiency as compared to the NIP. This may be because of the presence of complimentary binding sites in the adsorbent MIP as compared to NIP. This is due to the fact that NIP was synthesized in the absence of a template molecule. At the beginning, 2, 4, 6-TCP molecules were bound by the exterior surface of MIP, and the adsorption rate was fast. When the binding sites of the exterior surface reached saturation point, 2, 4, 6-TCP molecules entered into the pores of MIP and were bound by the interior surface of the MIP particles [8-11].
3.8. Effect of initial Concentration
In this study, the rebinding of the template was carried out at Various concentration of 2,4,6-TCP (20, 25, 30, 35, 40 ppm). The results depicted in Fig. (8) show that the rebinding efficiency for 2,4,6-TCP by MIP increases as the equilibrium concentration increases (20-30 ppm). The maximum rebinding efficiency (92.26%) was observed at 30 ppm. This may be due to the presence of a greater number of available sites in the MIP. Further increase in concentration (35-40 ppm) decreased the rebinding efficiency of MIP. This indicated that the all the binding sites were fully saturated at 30 ppm and with the increase in concentration, the available number of binding sites decreased and hence rebinding efficiency also decreased. This may be because of the accumulation of template molecules on the surface of the adsorbent [8, 9, 35]. This finding is in good agreement with the reported research suggesting that the adsorption of phenol by adsorbent increases with an increase in the initial concentration of phenol which indicates that there are plenty of accessible binding sites available for phenol adsorption until it reaches equilibrium. After that, it decreases slightly due to saturation of the adsorbent [8-11, 36, 37].
3.9. Effect of Dosage of MIP
The adsorbent dosage and surface available for the adsorption process can affect the efficiency of adsorption considerably [38]. In this study, a different amount of polymer dosage was used to observe the rebinding efficiency. Fig. (9) indicates that the rebinding efficiency increased (87.41%) with an increase in the dosage of polymer ranging from 100-200. This trend is expected with the fact that the number of binding sites available for the template molecules increases with the increase in the adsorbent dosage, and thus more template was attached with the MIP. Therefore, this increased the rebinding efficiency until reaching the saturation point [7, 8, 39-41]. Furthermore, an increase in polymer dosage ranging from 300-500 mg decreased the rebinding efficiency. This may be due to the aggregation or interparticle interactions with the polymer particles. This indicated that the number of available binding sites decreased due to the aggregation of polymer particles. The increase in the rebinding efficiency can be explained by the fact that, when the mass of the adsorbent is increased, the specific surface area and the efficiency of adsorption increase and hence the elimination of the 2, 4, 6-TCP.
3.10. pH Study
The pH of the solution is one of the most important parameters that can change the chemical properties of both absorbing and adsorbing materials. The change in the solution pH can alter both aqueous chemistry and surface binding sites of the adsorbent [42, 43]. The pH also affects the surface properties of the sorbent, i.e., surface charge of the cells used as sorbent [44, 45]. In this study, rebinding efficiency was observed in acidic, neutral and in basic conditions. The results depicted in Fig. (10) show that the higher efficiency (85.95%) was observed at pH 7 as compared to acidic and basic conditions. This may be due to the change in functionality both in acidic and basic conditions of the template. This change in interaction sites on template complementary with the polymer matrix decreased the rebinding efficiency. This is also because at lower pH, higher H+ ions concentration competes for hydrogen bond formation between hydrogen molecules in 2, 4, 6-TCP and similarly with the COOH group of MAA monomer, at the basic pH, hydroxyl ions interrupt the hydrogen bonding.
3.11. Selectivity Test
In this study, melamine was used as a competitive template, a structural analogue to 2,4,6-TCP. Table 4 shows the results of the selectivity of MIP towards the template. The results clearly indicated that the adsorption capacity of MIP towards 2,4,6-TCP is higher than NIP. This indicated the specific binding of the template by MIP. From the results, it is also clear that MIP particles have higher adsorption capacity towards 2,4,6-TCP as compared to melamine. The imprinted sites generated in the MIP have the ability to recognize target templates through their size, shape and functional group distribution [45]. However, the NIP only adsorbs 2,4,6-TCP and melamine on the surface due to a lack of imprinted sites in the polymer matrix. A good relative selectivity coefficient for the 2,4,6-TCP was achieved in this study.
3.12. Application
The 2,4,6-TCP has been used extensively as an antisepticc, a pesticide for wood, as leather, glue preservation, and as an anti-mildew treatment. Its high toxicity, carcinogenic properties, and persistence in the environment have led to an idea to synthesize MIP for removal of 2,4,6-TCP from human blood serum and environmental water to protect from its hazardous effects. The MIP has successfully been used to extract/remove 2,4,6-TCP (Table 5) from spiked blood serum (88.57%) and river water (94%) samples.
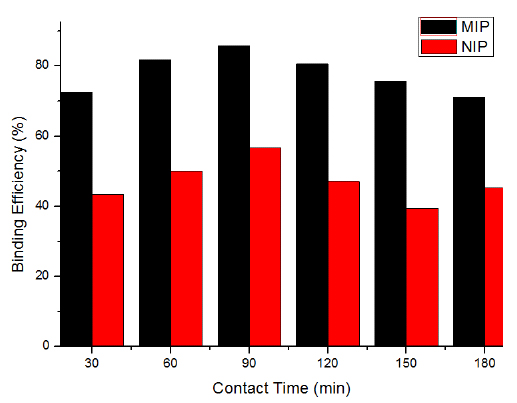
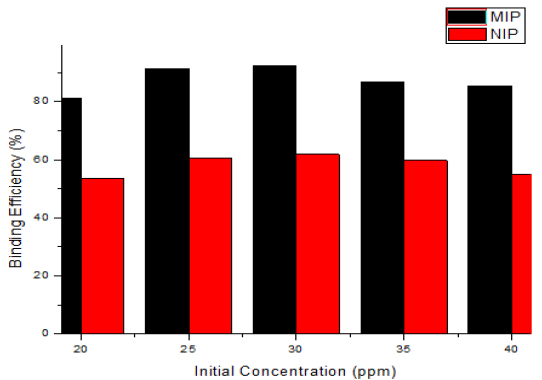
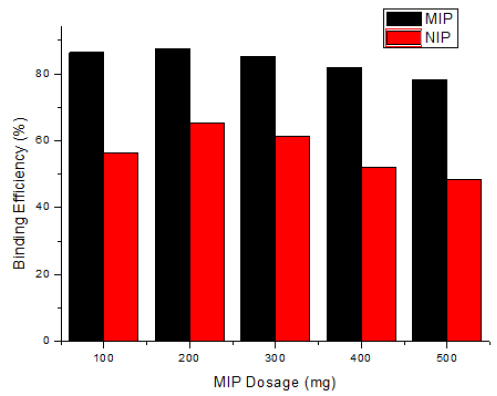
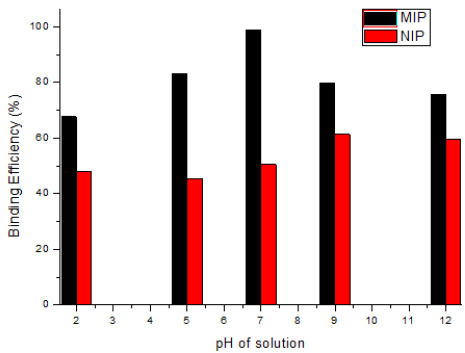
Polymer | Template (mmol) | Monomer(mmol) | Cross-linker(mmol) |
---|---|---|---|
MIP1 | 1 | 3 | 10 |
MIP2 | 1 | 4 | 10 |
MIP3 | 1 | 5 | 10 |
NIP | - | 5 | 10 |
S.no. | Parameters | Variation in parameter | Constant parameters |
---|---|---|---|
1 | Contact time | 30,60,90,120,150,180 min | Temperature 300 K, Agitation speed 250 rpm, Adsorbent dose 200 mg, Concentration 30ppm, PH 7 |
2 | Different initial concentration | 20, 25, 30, 35, 40 ppm | Temperature 300 K, Agitation speed 250 rpm, Contact time 90 min, Adsorbent dose 200 mg, PH 7 |
3 | Different dosage | 100,200,300,400,500 mg | Temperature 300 k, Agitation speed 250 rpm, Contact time 90 min, pH 7, Concentration 30 ppm |
4 | pH | 2,5, 7 ,9,12 | Temperature 300 K, Agitation speed 250 rpm, Contact time 90 min, adsorbent dose 200 mg, and Concentration 30 ppm. |
Properties | Magnitude MIP | Magnitude NIP |
---|---|---|
Surface area (m2/g) | 19.931 | 7.961 |
Average Pore Radius (Å) | 3.9679 | 1.2453 |
Total Pore volume (cc/g) | 3.954 | 2.653 |
Template | KD (MIP)(mLg−1) |
KD (NIP) (mLg−1) |
k sel | k |
---|---|---|---|---|
2, 4, 6-TCP | 7.22 | 3.33 | 2.7 | 2.30 |
Melamine | 2.83 | 2.6 | 1.18 |
- | - | - | MIP 3 | - | - | NIP | - |
---|---|---|---|---|---|---|---|
Samples |
Amount of 2,4,6-TCP added (µg/mL) |
Amount of 2,4,6-TCP found (µg/mL) | Recovery (%) | RSD (%) | Amount of TCP found (µg/mL) | Recovery (%) | RSD (%) |
Blood Serum | 30 | 26.4 | 88 | 0.26 | 15 | 50 | 0.58 |
River Water | 30 | 28.2 | 94 | 0.24 | 13.8 | 46 | 0.88 |
CONCLUSION
The 2, 4, 6-trichlorophenol imprinted polymer prepared in this study also showed good selectivity for 2, 4, 6-trichlorophenol against the competing structural analogue, melamine. An MIP for 2, 4, 6-trichlorophenol was synthesized using MAA as a functional monomer, and DVB as a crosslinker via precipitation polymerization method. The MIP was characterized by Fourier transform infrared spectroscopy (FTIR), scanning electron microscopy (SEM) and energy dispersive X-rays (EDX). Different experiments with parameters including the effect of pH, different initial concentrations, different MIP dosages, contact time, and agitation rate were conducted. The precipitation polymerization was found useful for the production of polymer particles of uniform shape and size. The produced MIP particles had a greater surface area, pore size and pore volume as compared to NIP. The optimized MIP was successfully used for the extraction of 2,4,6-TCP from blood serum (88.57%) and river water (94%). The synthesized MIP could be used as a potential material for the solid-phase extraction and as a stationary phase material for chromatographic separations.
CONSENT FOR PUBLICATION
Not applicable.
AVAILABILITY OF DATA AND MATERIALS
Not applicable.
FUNDING
None
CONFLICT OF INTEREST
The authors declare no conflict of interest, financial or otherwise.
ACKNOWLEDGEMENTS
Authors are thankful to the Faculty of Resource Science and Technology, UNIMAS, Sarawak for providing necessary research facilities.