All published articles of this journal are available on ScienceDirect.
Application Of Seeding Crystallization as a Pre-Treatment Stage for The Reduction of Scaling Tendency of Seawater Feed to Thermal Desalination Units
Abstract
Objective:
The fouling inhibition in seawater desalination and scale control using crystallization with calcite seeds is evaluated experimentally in this study.
Methods:
The growth kinetic parameters are determined experimentally, correlated and discussed at different operating conditions. Supersaturation levels represent driving force behind the growth of crystals, which is influenced directly by seawater pH values and temperatures.
Results:
Results indicate that the initial pH value of seawater must be controlled to be in the range (8-9) and calcite seeds will not have the potential to start the growth process in seawater at the normal pH (7.36). The growth kinetic parameters are determined from the measured desupersaturation curves.
Conclusion:
It is found that the growth process of calcite is controlled by the surface integration step. The growth rate of calcite increases with increasing temperature and seeding ratio (up to 1 g/L), while it decreases with increasing the salinity of seawater.
1. INTRODUCTION
Formation of scaling deposits of sparingly soluble salts on the outer surfaces of hot pipes in Multi Effect Distillation (MED) units represents a severe problem in seawater desalination. These mineral deposits form hard and strongly adhering layers on the metallic surfaces [1-3]. Calcium carbonate is the main component (98% of the layer) at a temperature level less than 90 °C and can be crystallized in three different polymorphs: calcite, aragonite and vaterite. Calcite is the least soluble form and its crystallization on the hot surfaces is enhanced by the decrease of its equilibrium solubility at elevated temperatures in the metastable seawater. The formation of such layers reduces the heat transfer and therefore causes an increase in energy costs with the accompanying problems such as the danger of overheating, unscheduled shutdown of the unit and corrosion, which leads to deterioration of the metallic pipes of the heat exchangers.
The scaling/fouling inhibit and control in seawater desalination and can be attained successfully through the applications of antifouling agents, where such polymer additives are added constantly with metastable water, which in return results in significant environmental and economic issues that can be problematic later on, in addition to contributing to increased total cost if desalination.
A proposed alternative is separating the scaling materials from seawater by a seeding procedure in a crystallization process. The principle of the seeding technique is based on separating of the sparingly soluble scaling of seawater materials through the process of crystallization at surface of the seed crystals. In the literature, there are many studies (many years back) that reported experimental investigations [4, 5], that tried to find whether it is possible to apply technology of seeding on scale inhibition over an industrial scale. Weth and Coury [6] studied the application of seeding methods in falling films plate evaporators for scale inhibition.
Several experimental investigations were conducted by Jones on horizontal pipes evaporators [7] and Rostein and Weinberg [8], which explained the positive findings. In applications of seeding technology, only a low number of studies addressed tubes of horizontal configurations and the majority of reports deal with vertical falling film evaporators and enforced circulation. The researcher was encouraged by scale control results through seeding inside heat exchangers to implement seeding technology on membrane technology [9].
The objective of the study is to contribute to improving scale of control and scale inhibition processes inside thermal desalination plants by seeded crystallization. Seeded batch experiments are conducted to attain the growth kinetic parameters for calcite seeds when growing in seawater. Kinetics of growth is determined by measuring de supersaturation curves in a seeded batch crystallizer under controlled conditions. The factors influencing the kinetics of growth, such as seed type, seeding ration, temperature, supersaturation, pH, and mixing were measured at different salinities of seawater. Calcite seeds are tested as seeds in artificial seawater.
2. THEORETICAL
Rate of growth for calcite in seawater is mainly dependent on supersaturation level. The supersaturation (Ω) for calcium carbonate is given through this equation

The terms [Ca2+] and [CO32-] depict the concentration of calcium and carbonate ions in seawater (mol/kg seawater), respectively, and Ksp is the solubility product constant of calcium carbonate in seawater [10]. Consequently, KSP has units of mol2/kg2 seawater. Seawater is supersaturated with calcium carbonate only when the value of Ω is more than one, which is the case at its normal salinity and temperature. However, the level of supersaturation is very small and the growth process will take longer time to achieve a measurable or noticeable growth, unless certain measures are taken to increase the driving force for crystal growth which can be attained by controlling the supersaturation in seawater with respect to calcium carbonate.
Several reactions govern supersaturation of calcium ions and carbonate during crystal growth of calcium carbonate in seawater, which happens during crystal growth in seawater. Such reactions produce a carbonate system in the seawater, this is summarized in Fig. (1).
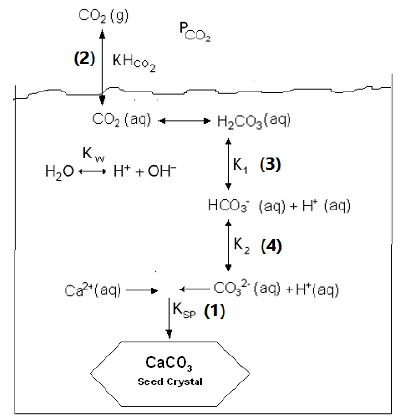
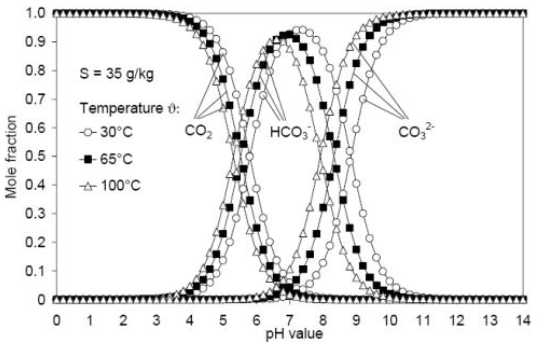
As shown in Fig. (1), in supersaturated seawater, growth process of calcium carbonate seeds results directly from ion association of carbonate (CO32-) ions and existing calcium (Ca2+) ions to form solid CaCO3 based on reaction 1. The ratio of bicarbonate/carbonate in sea water is affected by a series of equilibrium reactions 2, 3 and 4 (Fig. (1)).
Values of equilibrium constants Ksp, KHCO2, Kw, K1 and K2 can be estimated as a function of Temperature (T) and salinity (S) using empirical relations in literature. The knowledge of the liquid-liquid and gas-liquid equilibrium in seawater can be used to determine carbonate ion concentration and, the force behind the growth of crystal for calcium carbonate. In seawater, the concentration of carbonate ion depends on the pH value, temperature and salinity as reported by Millero [7], Fig. (2).
The results shown in Fig. (2) indicate that at constant pH, the mole fraction of CO2 decreases with increasing temperature, whereas the mole fraction of CO32- increases. As for values of pH no more than 7, mole fraction of HCO3- elevates for higher temperatures. However, pH values exceeding 7.5 show less values of HCO3- mole fraction and higher values of mole fraction of carbonate (CO32-) ions. As shown in the figure, pH values exceeding 7.5 will lead to an increase in CO32- and a decrease in HCO3-, accordingly, there will be a consequent increase in the thermodynamic force for the growth of calcine crystals in seawater.
In a closed seeded batch crystallizer, (CO32-) decreases due to the crystal growth of calcium carbonate seeds in the metastable seawater. This is accompanied by a pH-decay as can be understood from the following equation:
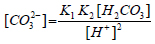
Where (H2CO3) and (H+) are the concentrations of the carbonic acid and hydronium ion in seawater in mol/kg seawater. K1 in equation 2 is the first thermodynamic dissociation constant of carbonic acid to bicarbonate ions in seawater (mol/kg seawater) whereas K2 is the second thermodynamic dissociation constant of carbonic acid to bicarbonate ions in seawater [7]. If the decrease in pH (decease in [CO32-]) over a certain period of time dt is attributed to the crystal growth, it can be written:

Where is corresponding to the growth rate of the seeds.
The growth rate data (Rm) can be fitted as a function of supersaturation (Ω) according to the following power law relation:

Where KR is the overall rate coefficient which includes both diffusion and surface integration steps and (n) is the overall growth order of seeds. The overall rate coefficient KR is usually temperature dependent according to the Arrhenius equation.
3. EXPERIMENTAL SECTION
As per the data of Millero [10], and for the entire set of experiments, seawater was prepared according to artificial standards and with salinities of 45 g/kg and 35 g/kg. Measurements were carried out in a crystallizer with specific specifications, given that it was closed tightly and jacketed and agitated with a capacity of 500 cm3. In addition, control of temperature (± 0.01 K) was conducted inside the crystallizer through the connection of crystallizer jacket to water feed taken from a programmed thermostat (Julabo-F32). A pH meter was installed to monitor the variation of the pH value (± 0.01).
400 mL of artificial seawater was added, which was prepared at specific salinity for bath crystallizer within a specific temperature and pH, which led to measuring the growth kinetics. The modifying of seawater pH took place by using KOH solid and HCI solution concentrated. Dried rewashed seeds with fine size (0-10 µm) were added to seawater after it reached stable temperatures and pH. Calcite seeds were used for the test. Rate of decay for pH was recorded as a function of time, then the curves of decay for pH were implemented to calculate de supersaturation rate over time, given that growth rates are a function of supersaturation.
4. RESULTS AND DISCUSSION
4.1. Calcite Growth Kinetics (Salinity 45 g/kg), Effect of Temperatures
The measured pH decay as a function of time at different temperatures is displayed in Fig. (3). The results presented here are related to the growth of calcite seeds in seawater at a salinity of 45 g/kg. As presented in Fig. (3), the rate of pH decrease is clearly faster with increasing temperature. The calculated growth rates as a function of supersaturation are shown in Fig. (4). The results presented in Fig. (4) indicate that the growth rate of calcite increases with increasing temperature. Higher temperatures will lead to a decrease in the solubility of calcium carbonate and therefore, the value of Ksp will decrease. Consequently, the level of supersaturation will be higher at elevated temperatures. Moreover, the temperature is a key parameter affecting the carbonate systems and the equilibrium constants (K1, K2 and Ksp), which has a direct influence on the amount of carbonate ions in seawater. The kinetic parameters of growth are evaluated by the exponential fitting of the results displayed in Fig. (4) according to Eq. 4. The results are summarized in Table 1.
T [°C] |
KR [g/m2.s] | n [-] | R2 (eq. 4) |
---|---|---|---|
60 | 2 × 10-6 | 2.0541 | 0.9573 |
50 | 2 × 10-6 | 1.895 | 0.9849 |
40 | 2 × 10-6 | 1.7229 | 0.9998 |
30 | 9 × 10-7 | 1.7672 | 0.9384 |
Results shown in Table 1 indicated that as the temperature increases, the growth rate order increases at salinity value of 45 g/kg. A higher value of rate growth rate order (n) suggests that the integration step to the seed surface turns a more dominant growth step at elevated temperatures. The parameter of growth rate constant (KR) does not change within range of temperature between 40 °C and 60 °C, while it changes with temperature ranges between 30 °C and 40 °C.
4.2. Effect of Salinity Over Calcite Growth Rate
The decay of pH is recorded during the growth of calcite seed crystals in synthetic seawater at various salinities. The measured growth rate versus supersaturation at the salinities 35 and 45 g/kg are depicted in Fig. (5).
The results presented in Fig. (5) indicate that a faster growth rate is measured at the lower salinity and the difference increases at higher supersaturation. The higher salinity the seawater has, the more dissolved species and, therefore, lower diffusion rate of the calcium and carbonate ions can be found. Also, increasing the salinity will increase the number of ions that can be adsorbed on the growth sites of crystal surfaces of calcite such as magnesium ions. This will contribute to a reduction of the growth rate of calcite at higher salinities.
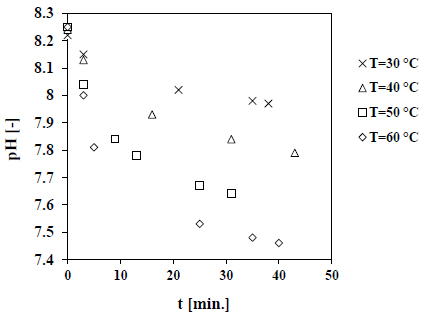
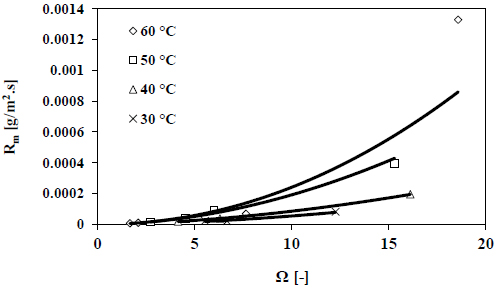
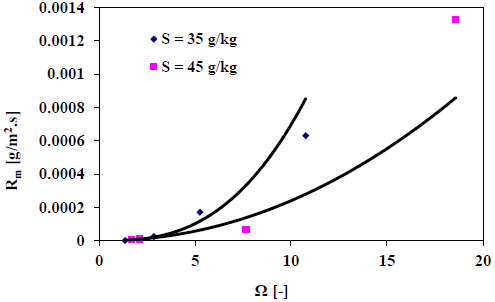
4.3. Stirrer Speed Effect
Fig. (6) shows the recorded decay of pH for various stirrer speeds over time which is used to calculate the growth rate at different supersaturation levels presented in Fig. (7).
The results presented in Fig. (7) show a minor effect of stirrer speed on the growth rate of calcite. This indicates that not the diffusion step but the surface integration step is the controlling step of the growth process of calcite in seawater. Also, the stirrer speed has a minor effect on the equilibrium reactions of the carbonate system in seawater which is only dependent on pH and temperature.
4.4. Calcite Growth Kinetics in Seawater, the Ratio of Seeding Effect
Measurements of pH decay rates were conducted in a batch crystallizer that is seeded at different seeding ratios are presented in Fig. (8). All the measurements were conducted at a fixed temperature (50 °C) and salinity (35 g/kg). Fig. (9) shows the calculated growth rates as a function of the seawater supersaturation with respect to calcite.
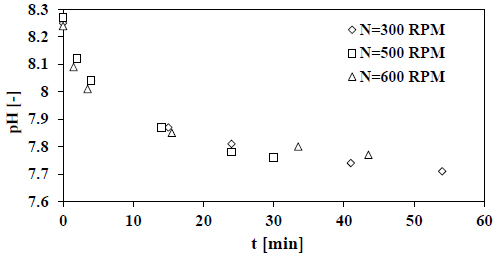
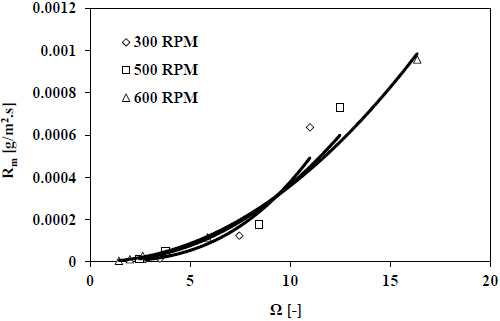
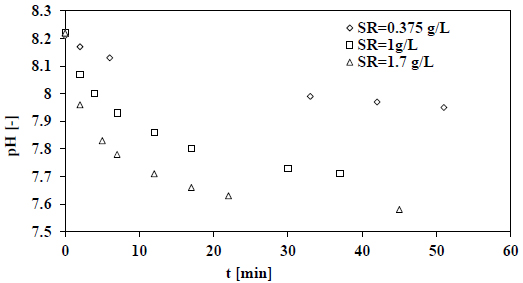
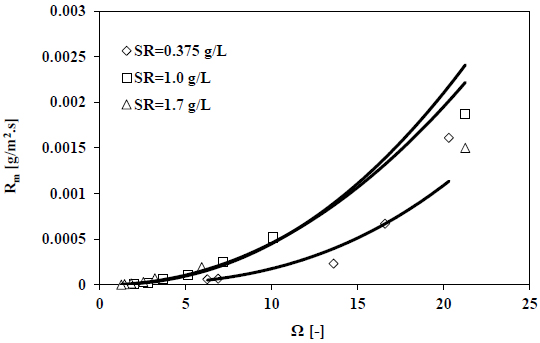
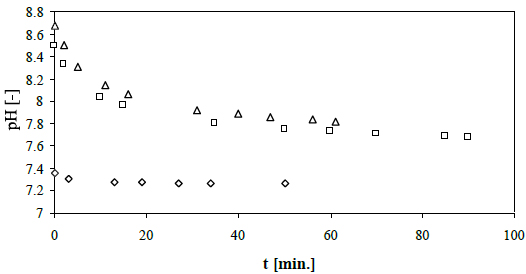
From Fig. (9) above, the results suggested that increasing the ratio of seeding from 0.375 to 1 g/l will lead to an increase in the rate of growth of calcite. Nonetheless, there is a negligible effect on the rate of growth when the ratio of seeding increases further. Moreover, at higher seeding ratios, the rapid rate of mass deposition is caused by the enlarged existing surface area of the crystal growth.
4.5. Influence of the initial pH in seawater on initial growth rate.
Experiments were conducted to test how the initial values of pH in seawater influence the initial rate of pH decay amid the crystal growth for the seeds under definite conditions of temperature, stirrer speed, seeding ratio, and seeds type. The measurements of the pH decay rate, which indicates the initial seed growth rate at 50 °C for the normal seawater without adjustment of initial pH (pH = 7.36) and at the adjusted higher pH values are shown in Fig. (1).
The experimental data which are depicted in Fig. (10) indicate that seeds of calcite lack the ability of starting growth processes at pH (7.36) in seawater, giving that the increments of initial value of pH above 8.4 (see the upper data points of Fig. (10) will lead to faster initial pH decay, thus faster initial growth rates. As a result, to achieve an appreciable growth of seeds of calcite inside the seawater, therefore, the values of the pH must be adjusted to be higher than or at least 8.0. These results are in good agreement with the theoretical calculations shown in Fig. (2).
CONCLUSION
Crystallization with seeding can be implemented successfully to control the scaling process thermal seawater desalination units if the process parameters and the kinetic data are well understood. This study represents the starting stage to replace the already existing methods based on dosing anti-scaling chemicals with a reliable, economical and low environmental impact method based on physical separation of scaling minerals by crystallization. Further studies to develop its industrial application are recommended.
CONSENT FOR PUBLICATION
Not applicable.
AVAILABILITY OF DATA AND MATERIALS
Not applicable.
FUNDING
None.
CONFLICTS OF INTEREST
The authors declare no conflict of interest, financial or otherwise.
ACKNOWLEDGEMENTS
Declared none.