All published articles of this journal are available on ScienceDirect.
Fast and Facile Voltammetric Detection of Acetaminophen at Poly (DL-phenylalanine) Modified Dysprosium-Copper Oxide Nanoparticle/Carbon Composite Paste Electrode
Abstract
Introduction:
The voltammetric sensing of Acetaminophen (AN) using modified Dysprosium Copper Oxide (DyCuO) Nanoparticles (NP) mixed Carbon Paste Electrode (MCPE) was successfully developed.
Methods:
The modification of bare NPMCPE was achieved by the polymerisation of DL-Phenylalanine (DLPA). The electroanalysis of the AN was achieved by utilizing the Cyclic voltammetry (CV) approaches. The crystallographic nature of the nanoparticle was studied via X-ray Powder Diffraction (XRD) technique. The surface morphology and electrochemical feature of the prepared electrode were evaluated by Field Emission Scanning Electron Microscopy (FE-SEM) and Electrochemical Impedance Spectroscopy (EIS) techniques.
Results:
The modified sensor exhibited an excellent electrocatalytic activity towards the electroanalysis of the AN. Several aspects, such as the number of polymerisation cycles, variation of pH, and the impact of scan rate were investigated in 0.2 M supporting electrolyte (pH 7) at a sweep rate of 0.1 Vs-1. The suggested sensor shows a very low detection limit (11.95×10-8 M) with a linear range of 2.0 to 50.0 µM, which exhibits excellent sensitivity.
Conclusion:
The stable and reusable sensor was applied for the estimation of AN in the tablet sample. Thus, P(DLPA)MNPMCPE was utilized as the most capable sensor for the voltammetric detection of AN.
1. INTRODUCTION
AN, also referred to as paracetamol, is an acylated aromatic amide, which was first introduced as an analgesic drug in the field of medicine by Von Mering in 1893 [1]. AN is a widely used analgesic drug which is used for the treatment of various aches, such as aches of muscle, aches in the tooth, body pains, headache and backache, etc [2]. This drug is also advised to reduce cough, common cold, and even to reduce fever; AN is one of the major drugs for cold remedies, fever, and is sold under various pharmaceutical drug products [3, 4]. AN acts as an important acetyl salicylic acid alternative due to its antipyretic and analgesic effects [5]. AN is safe if taken in appropriate doses for the patient, and it is less harmful in nature as excessive inactive metabolites are excreted along with urine. However, its excessive intake may lead to over dosage, and this may affect the body [6, 7]. Thus, a cost-effective, sensitive and accurate method to determine AN in pharmaceutical samples has been a crucial area of research for the benefit of public health.
Until now, various analytical methods have been used in the detection of AN, such as UV Spectrometry [8], Titrimetric Determination [9], High Performance Liquid Chromatography Method [10], Spectrofluorometry Method [11], and Flow injection chemiluminescence [12]. Although these methods provide high sensitivity and accuracy, some of these methods require high cost and long time analysis, so it is necessary to detect the AN by electrochemical methods. In recent years, electrochemical sensors have attracted greater attention due to their simple setup and more sensitive response towards analyte detection, and within a short time, they provide excellent repeatability and stability [13-17].
Among various kinds of electrodes, carbon paste electrodes are extensively used due to their low cost, easy development, simple modification, easy renewability and good sensitivity [18-21]. Among several electrode modification methodologies, such as immobilization, modification in situ, electropolymerisation, dissolution, bulk modification, impregnation, etc., the electropolymerization method has been incorporated due to its simple modification process, uniform coating capability on the surface and potential towards excellent film formation; the modifier has the capability to enhance the electron transfer rate and build strong adherence for the surface of the electrode [22-24]. Amino acids are the biocompatible electroactive monomers that possess the ability to form their own functional groups, hence electropolymerization of amino acids is very much crucial. DL-Phenylalanine is an amino acid which can be easily electropolymerized on the surface of the constructed electrode [25, 26].
In this research article, voltammetric analysis of the AN was achieved by constructing the poly DL-Phenylalanine on the dysprosium copper oxide nanoparticles combined carbon paste electrode. The modified electrode exhibited boosted electrocatalytic activity compared to the bare electrode and yielded a low detection limit. The electrochemical response of the AN was assessed by using the CV and differential pulse voltammetry (DPV) methods. After a thorough literature survey, it has been observed that no works are reported on P(DLPA)MNPMCPE for the detection of AN. Finally, the viability of the projected sensor was evaluated by detecting the AN content in the paracetamol tablet.
2. MATERIALS AND METHODS
2.1. Instrumentation
The voltammetric measurements were recorded by employing the electrochemical work station CHI-6038E (CH. Instruments, USA) with the three electrode compartment, and the analyser was connected to a personal computer for the control and storage of the data. BNPCPE, P(DLPA)MNPMCPE served as the working electrode, the platinum electrode was kept as an auxiliary electrode, and an aqueous saturated calomel electrode was employed as a reference electrode. The different pH solutions were prepared using the EQ-160 digital pH device. The double-distilled water was acquired by VITSIL-VBSD/VBDD water purifier instrument. FE-SEM (operating in keV) data for the surface study of P(DLPA)MNPMCPE and BNPCPE were received from DST-PURSE Laboratory, Mangalagangotri, Mangalore University, India. XRD data (Co Kα radiation) of the nanoparticle was collected from JSSSTU Mysore, India.
2.2. Chemicals and Reagents
AN was acquired from Tokyo Chemical Industry Company Ltd., Japan. Graphite powder and Silicone oil were procured from Nice Company, India. Monosodium hydrogen phosphate (NaH2PO4), Disodium hydrogen phosphate (Na2HPO4) and DL-Phenylalanine were obtained from Molychem, India. The chemicals that were utilized in this experiment were of analytical grade and used without any purification. The AN stock solution (25×10-4 M) and DL-Phenylalanine (25×10-4 M) were prepared in distilled water. Appropriate amounts of 0.2 M NaH2PO4.H2O and 0.2 M Na2HPO4.H2O were mixed for the experiments at proper pH, and used as the supporting electrolytes. All the experiments were conducted at the lab temperature (25 ± 1°C).
2.3. Synthesis of DyCuO Nanoparticles
DyCuO nanoparticle was synthesized by the simple co-precipitation method. Briefly, 50ml of 0.05 M (DyNO3)2 and 50 ml of 0.1 M Cu(NO3)2 were prepared using triple distilled water and mixed in a 1:1 ratio to yield a homogeneous mixture. Subsequently, 0.1 M NaOH solution was added dropwise to attain an approximate pH of 10 until the precipitate has been formed. The precipitate was kept for magnetic stirring for about 3 to 4hrs at 80°C. The resultant precipitate was washed with distilled water and ethanol several times and dried in a hot air oven. Finally, the solid product was heated in a muffle furnace at 650°C for 6 hrs and was used for further experimental procedures.
2.4. Fabrication of the Electrode
A homogeneous paste was prepared by blending the graphite powder, DyCuO NP and silicone oil in the proportion of 60:10:30 (w/w) by grinding it in an agate mortar. A Teflon tube of 3 mm diameter was taken, which consisted of a copper wire for the electrical bonding, and the paste thus obtained was tightly packed into the crater of the Teflon tube; smoothening was done by rubbing the surface of the tube on the filter paper, and thus, an unmodified electrode has been obtained as a result. The modified electrode was constructed by the method of electropolymerization of DL-Phenylalanine in supporting electrolyte of optimum pH, which was swept continuously for 10 cycles on the surface of the unmodified electrode. Finally, the electrode was rinsed with distilled water to remove the physically unadsorbed DL-Phenylalanine. The electrode was coined as P(DLPA)MNPCPE, and the modified electrodes were utilized for the electrochemical detection of AN throughout the experiment.
3. RESULTS AND DISCUSSION
3.1. Electropolymerisation of DL-Phenylalanine on the bare Electrode
Among various modification methodologies employed, electropolymerization is a simple method for altering the CPE’s electrocatalytic activity. Hence, electropolymerization of DL-Phenylalanine was performed in 0.2 M supporting electrolyte of pH 7.0 at a constant sweep rate of 0.1 Vs-1, keeping the potential window at 0 V to 1.6 V by running 20 continuous segments of CV (Fig. 1a). As can be inferred from the Cyclic Voltammograms (CVs), the layer of the electropolymerisation intensifies by escalating the CV cycles signifying that the poly DLPA covers the BNPCPE film. Furthermore, in order to gain the highest response of the analyte, the electropolymerisation was conducted by differing polymerization cycles from 5 to 20. The bar graph of peak current with respect to electropolymerization cycles (Fig. 1b) confirms the enhancement of peak current till the 10th cycle. As we moved beyond the 10th cycle, the peak current diminished gradually due to the electron transfer of AN at P(DLPA)MNPMCPE in an inappropriate proportion. Hence, the number of sweep cycles for the electropolymerization was kept constant for 10 cycles.
3.2. XRD and FE-SEM Analysis
To analyse the surface morphology of the designed electrode, FE-SEM technique was employed for the prepared electrodes. The captured FE-SEM images for the different electrodes are displayed in Fig. (2). The flakes of the graphite and nanoparticles have been observed from the FE-SEM images of the BCPE (Fig. 2a) and DyCuO nanoparticles (Fig. 2b and c) portrays the FE-SEM of BNPMCPE containing both flakes and particles. The FE-SEM photos of P(DLPA)MNPMCPE reveal that poly DLPA covers all the surface of BNPMCPE. Thus, the FE-SEM analysis indicates that the bare electrode was successfully modified by electropolymerisation. The crystalline nature of the material was analysed using XRD. The XRD (Fig. 3) of the DyCuO nanoparticles was performed which depicted the polycrystalline pattern of the CuO phase. The distinctive diffraction peaks at 2θ 29.320, 35.770, 39.010 and 48.690 were found to correspond to the planes of DyCuO, respectively.
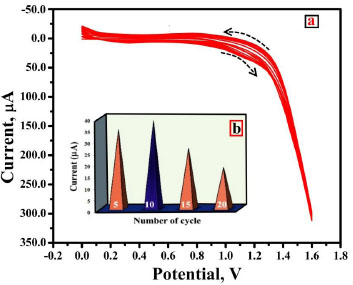
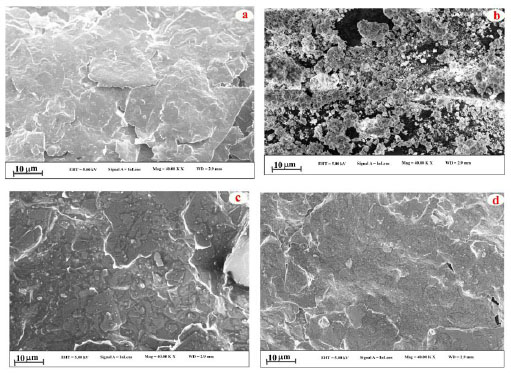
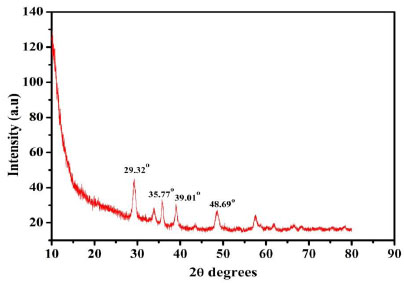
3.3. Electrochemical Characterisation of the Proposed Electrode
CV is an influential tool that indicates the material catalytic behaviour towards the redox reaction of the molecule. Fig. (4) shows the CVs of 0.1 mM K4Fe(CN)6 in 0.1 M KCl as a buffer solution on the surface of BNPMCPE (curve a) and P(DLPA)MNPMCPE (curve b) at a sweep rate of 0.1 Vs-1. Here, we observed that the bare electrode provided a lower oxidation peak current compared to the modified electrode due to the effect of the active surface area. The calculation of active surface area was based on the following Randles–Sevcik relation (Eq. 1),

Where, A is the active surface area of the electrode, n is the number of electrons, C is the concentration of the analyte, D is the diffusion coefficient, and the physical meaning of other terms is already known [27]. The calculated active surface area for BNPMCPE and P(DLPA)MNPMCPE is 0.05 cm2 and 0.02 cm2 for modified and bare electrodes, respectively.
3.4. EIS Characterization of Electrodes
The property of the surface of the electrode after modification was studied by using EIS. The impedance measurement was carried out at a frequency of 1mM of K4Fe(CN)6 with 0.1 M KCl as the supporting electrolyte. In the Nyquist plot, the semicircle found at a higher frequency referred to the restriction of electron transfer phenomenon and the linear section at a lower frequency range represented the diffusion electron transfer process. As can be noted from the Nyquist diagram shown in Fig. (5), the largest semicircle at the high frequency was observed for bare electrode with an Rct value of 9145 Ω. After modifying with polymer, the Rct value was found to minimise to a value of 206 Ω. This confirms that the P(DLPA)MNPMCPE film is capable of accelerating the electron transfer signifying good conductance of the electrode. In turn, the P(DLPA)MNPMCPE proved to have a catalytic effect on the voltammetric behaviour of the redox probe. The heterogeneous electron transfer rate constant (k0) can be determined by considering the obtained Rct values from the below Eq. (2),
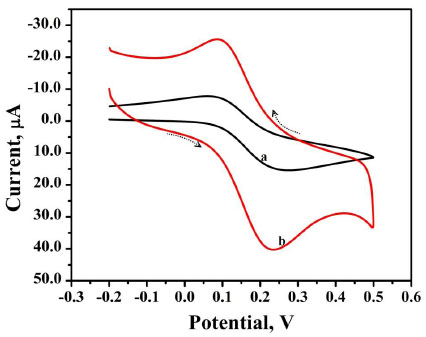

Where, n represents the number of electrons transferred, A depicts the area of the electrode, C is the concentration of the solution, and other symbols are already known [28]. The calculated k0 value for the modified electrode (2.5×10-5 cms-1) is higher than the bare electrode (1.4 × 10-6 cms-1), clearly signifying that the modified electrode promotes redox reaction better than the bare electrode.
3.5. Electrochemical Response of AN on BNPMCPE and P(DLPA)MNPMCPE
The voltammetric behaviour of 0.1 mM AN in 0.2 M buffer solution (pH 7.0) on BCPE (curve a), BNPMCPE (curve b) and P(DLPA)MNPMCPE (curve c) has been examined by the CV method at a sweep rate of 0.1 Vs-1 within the potential of -0.10 to 0.70 V. It can be seen from Fig. (6) that the P(DLPA)MNPMCPE shows excellent current response, in that the peak current was located at the peak potential of 0.340 V compared to BNPMCPE (0.420 V). This result signifies that the modified electrode reduces the overpotential with enhanced catalytic nature due to the influence of the high active surface area and anti-fouling property than the unmodified electrode. Additionally, the modified electrode did not give any of the redox peaks in the absence of AN in the supporting electrolyte, indicating that the response of the electrode is completely dependent on the mutual interaction of the AN and buffer solution.
3.6. Effect of pH
The pH has a distinct effect on peak current, peak potential, stability of analyte, and the shape of voltammograms in the voltammetric detection of bioactive molecules. So in order to optimize pH, the determination of AN was performed at P(DLPA)MNPMCPE in pH range 5.5-8.0 with a potential scan rate of 0.1 Vs-1, as depicted in Fig. (7a). The anodic current of AN was found optimum at pH 7.0, as represented in Fig. (7b); this pH was considered as the calibrated pH for the complete analysis of AN. It can be observed from the plot of Epa and Epcvs. pH (Fig. 7c) that as the solution pH increases, the basicity increases and the peak of AN shifts to a higher negative scale. The linear fit of Epa and Epcvs. pH is described by linear regression as


The slope 0.056 (experimental) is close to 0.059 (theoretical), so as per the Nernst equation, the electrochemical interface of AN at P(DLPA)MNPMCPE involves the same quantity of electrons and protons [29]. With the help of the obtained slope from Epc and Epa, the ratio of number of electrons was calculated by using the following formula,

where, m is the number of protons and n is the number of electrons [30]. The estimated m/n ratio was found to be 1.040 and 1.376; these values convey that the same quantity of protons and electrons was present in the redox reaction of AN at the prepared electrode.
3.7. Impact of Potential Sweep Rate
The impact of sweep rate from 0.1 to 0.30 Vs-1on the redox reaction of AN at the PDLPAMNPMCPE was evaluated in 0.2 M buffer solution at pH 7.0 having 0.1 mM AN, as documented in Fig. (8a). This, in turn, results in an increase of peak current against the change of sweep rate. A graph of the square root of sweep rate v/s Ipa is plotted, and it is depicted in Fig. (8b); the linearity is expressed using the following Eq. (6).
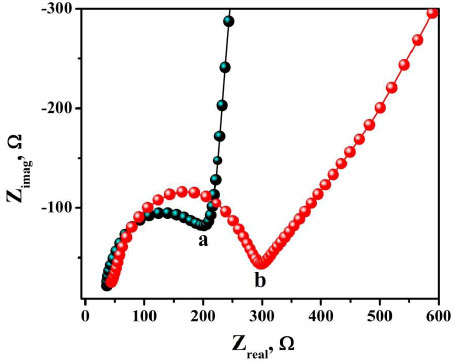
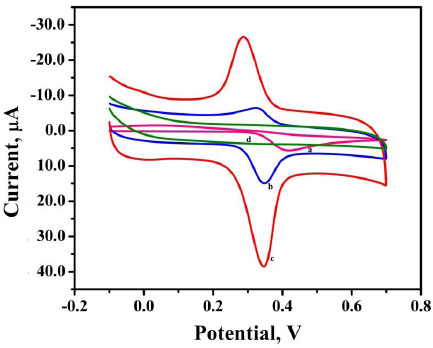
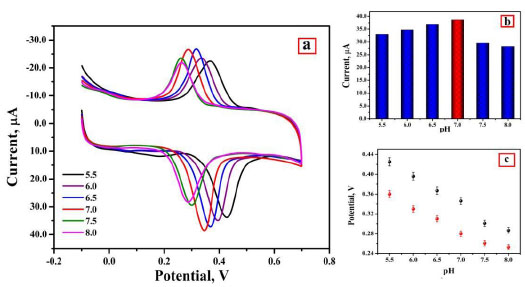
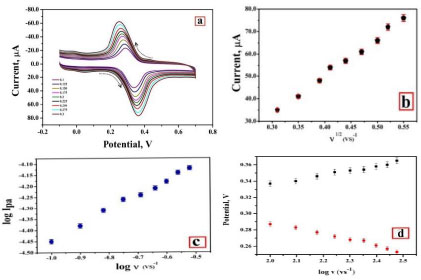

The electrode-analyte interface process (adsorption/diffusion) of AN has been investigated, and slope 0.61 obtained from the plot of log Ipa v/s. log v (Fig. 8c); thus, we can conclude that the process was diffusion-controlled [31].

The graph of Epa and Epc against log v (Fig. 8d) has been constructed; the fitted linear equation is represented as follows


By using the above-obtained regression equations, it can be assumed that the electrons participated in the redox reaction of the AN at the prepared sensor. The slopes of the graph of Epa and Epc against log v have been found equal to the subsequent formulas,


Here, n indicates transferred electrons, α is a charge transfer coefficient, and other symbols are commonly known [32]. The electrons transferred during the redox reaction of the AN are estimated as two by Eqs (10 and 11).
3.8. Analytical Curve and Figure of Merit
DPV was accomplished for the oxidation of AN by differing its concentration at P(DLPA)MNPMCPE between 2 µM to 50 µM under optimum conditions. The graph of the concentration of AN with respect to peak current (Fig. 9) has been constructed. As can be inferred from Fig. (9), as the concentration of AN increased, the current response also intensified with the linearity remaining up to 10 µM; further, the linearity deviated from 10 µM to 50 µM. The regression equation measured for the first linearity from the concentration ranging between 2µM to 10µM is as follows,

The limit of detection and limit of quantification of the P (DLPA) MNPMCPE have been assessed by Eq. (11),

Here, SD is defined as the standard deviation obtained for 5 blank measurements, and M is the obtained slope of the calibration curve [33]. The measured LOD and LOQ values were 1.19 × 10-7 M and 3.9 × 10-7 M, respectively. The LOD values obtained for various classical techniques and electrodes are shown in Table 1 [34-40]. This devised electrode was found to be efficient in contrast to the other reported electrodes.
Sl No. | Electrode | Rct | X2 | K0 |
---|---|---|---|---|
1. | BNPMCPE | 9145 | 5.42 × 10-4 | 1.4 × 10-6 |
2. | P(DLPA)MNPMCPE | 206 | 7.98 × 10-4 | 2.5 × 10-5 |
3.9. Reproducibility, Repeatability and Stability of Electrodes
The reproducibility, repeatability and stability of the electrodes were examined using CV by using 1 × 10-4 M AN in 0.2 M supporting electrolyte at optimum pH 7.0 with a scan rate of 0.1 Vs-1. Reproducibility of the proposed electrode was attained and examined by five repeated measurements with respect to five different P(DLPA)MNPMCPE, and the RSD value was observed to be 3.8%. Also, the repeatability of the proposed P(DLPA)MNPMCPE was carried out by varying the standard solution by keeping the electrode constant for 5 different measurements, and thus, we observed the RSD value to be 3.2%. The analyte solution was taken for 30 successful runs of CV to check the stability of the proposed sensor. Even after the 30 cycles, the prepared electrode did not lose its stability in sensing the analyte, and hence, its voltammetric activity remained unaltered. Thus, the entire outcome certifies that the prepared electrode has excellent repeatability, sufficient reproducibility and agreeable antifouling feature.
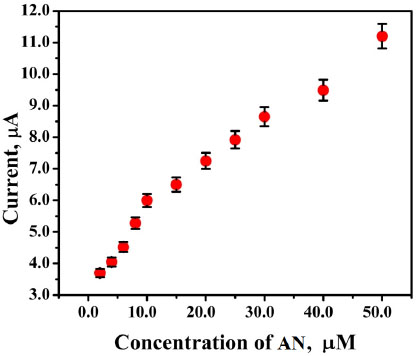
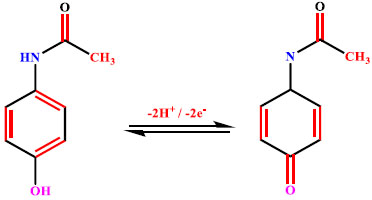
3.10. Simultaneous Detection of AN and DA
In biological samples, many interfering elements exist; thus, simultaneous separation of AN and DA at BNPMCPE and P(DLPA)MNPMCPE was conducted by DPV method with a scan rate 0.05 V/s, and the documented differential pulse voltammograms (DPVs) have been illustrated in Fig. (10). In the figure, the BNPMCPE identifies the AN and DA with low voltammetric responses, while the modified electrode gives two clear and characteristic peaks for AN and DA with a high current response. This shows that the equipped P(DLPA)MNPMCPE can be efficiently utilized for the instantaneous determination of AN and DA in a mixture. Hence, the suggested electrode possesses worthy selectivity for the detection of AN in the presence of DA Table 2.
3.11. Real Sample Analysis
To confirm the working capability and applicability of the projected sensor, the analysis of AN was accomplished in the tablet sample. The tablet containing AN content was procured from the drug store. The tablet was weighed, and using a mortar, the tablet sample was grinded and processed into a fine powder. The fine powder of the tablet was dissolved using distilled water and directly taken for CV scanning in 0.2 M supporting electrolyte. A good recovery range was observed from the current method. Tablet sample analysis and recoveries are displayed in Table 3.
Electrode Materials | Method | Linear Range(µM) | LOD (µM) | Reference |
---|---|---|---|---|
MCPE/PR | DPV | 0.7 - 100 | 0.53 | [34] |
Bi2O3/GCE PMMCNTPE |
CV CV |
0.5 – 1500 2.0 - 50 |
0.2 0.38 |
[35] [36] |
MIP | DPV | 1–4000 | 0.33 | [37] |
PIP/PGE | DPV | 5–500 | 0.79 | [38] |
Nafion/TiO2GR/GCE PASMCNTMGPE |
DPV DPV |
1–100 20-350 |
0.21 0.04 |
[39] [40] |
P(DLPA)MNPMCPE | DPV | 2.0 to 50.0 μM | 0.01 | This Work |
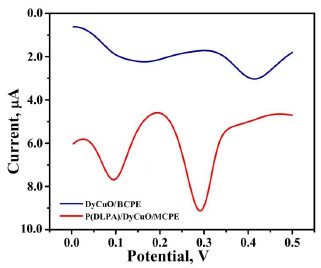
Sample | Concentration Added (µM) | Found Value (µM) | Recovery (%) |
---|---|---|---|
Tablet Sample | 20 | 20.13 | 100.6 |
30 | 30.32 | 101.1 | |
40 | 41.79 | 104.4 |
CONCLUSION
In summary, the sensing device based on modification by electropolymerisation of amino acid on DyCuO nanoparticle mixed with graphite paste electrode was constructed. The suggested electrode was magnificently employed for the detection of AN. The DyCuO nanoparticle was synthesised by an uncomplicated co-precipitation approach. The surface characteristics and resistance of the modified and unmodified electrode were evaluated by FE-SEM and EIS analyses. The crystallographic insights of the DyCuO nanoparticles were examined through the XRD technique. The developed P(DLPA)MNPMCPE provides excellent improvement in the voltammetric behaviour of AN in 0.2 M buffer solution at an optimum pH of 7 and at a sweep rate of 0.1 Vs-1. The sweep rate study concludes that the redox reaction of AN at the modified sensor was controlled by diffusion with an electron transfer of two. The projected sensor offers low LOD (11.95 × 10-8M) in a linear range of 2.0 to 50.0 μM with excellent sensitivity. Supporting parameters such as repeatability, reproducibility, selectivity and stability were found to be excellent of the proposed sensor in the case of AN sensing. Finally, the device was utilised for analysis of AN in the tablet sample. Overall, the designed sensor has been found efficient for routine analysis of AN in pharmaceutical analysis.
CONSENT FOR PUBLICATION
Not applicable.
AVAILABILITY OF DATA AND MATERIALS
Not applicable.
FUNDING
None.
CONFLICT OF INTEREST
The authors declare no conflict of interest, financial or otherwise.
ACKNOWLEDGEMENTS
Declared none.