All published articles of this journal are available on ScienceDirect.
Water Absorption Enhancement of Sodium Poly Acrylate and Poly(2-Acrylamido-2-Methylpropane Sulphonic Acid) Based Hydrogel Mixtures
Abstract
Introduction:
Hydrogels are hydrophilic polymers which are cross-linked to form three-dimensional structures, which can absorb, swell and retain huge amounts of water or aqueous fluids.
Objective:
This paper reports the preparation and characterisation of Poly(2-Acrylamido-2-Methylpropane Sulphonic Acid) (PAMPS) hydrogel with different crosslinking intensities.
Methodology:
2-Acrylamido-2-methylpropane sulfonic acid (AMPS) monomer was purchased from Alfa Aesar Company as reagent grade. It was used as received (>98% purity) without any further purification.
PAMPS hydrogel was prepared by free radical crosslinking solution polymerization of AMPS in water at room temperature under a nitrogen blanket in cylindrical glass tubes. The characteristics of the obtained PAMPS hydrogel were compared with those of commercial sodium polyacrylates hydrogel.
Results:
It was found that decreasing the crosslinker weight improved the absorbance capacity but to a limit. The suggested reasons were discussed. The mixture showed higher absorbance rate than PAMPS, and bigger absorbance capacity than sodium polyacrylates.
Conclusion:
This paper investigates the effect of crosslinker ratio on the swelling capacity of PAMPS. It was found that as the crosslinking ratio decreases, the porosity of the hydrogel increases, thus improving the swelling capacity.
1. INTRODUCTION
The hydrogel history is as early as in the 1800’s in the form of gels. Thomas Graham was the first researcher to study gels by using sol-gel chemistry to synthesis silica gel [1]. Since then, researchers focused on developing gels [2-4], Andrews hydrogels have been the centre of attention in recent years. Nowadays, researchers are interested in the preparation, characterisation, and applying hydrogels in various fields, particularly separation technologies [5].
The term ‘hydrogel’ was first used in 1894 to describe colloidal gels [6]. Then, in 1936, the first synthetic hydrogel was prepared by DuPont, which was poly(2-hydroxyethyl methacrylate) (PHEMA) [7]. However, the first application of PHEMA was only recognised in 1960 as contact lenses [8]. Since then, the use of hydrogels in medical applications has boomed. In fact, a new class of hydrogels was introduced known as bio-medical hydrogels (Vijay & Thakur, 2018). For example, the use of hydrogels in baby diapers was very popular in France and Germany in 1980 [9].
A hydrogel is neither a gel nor a polymer, in fact, it is a polymer gel. A gel is a soft solid material that has a three-dimensional structure with liquid-like properties, while a polymer is an extremely long molecular chain of repeated smaller units called monomers. Consequently, a hydrogel is a three-dimensional structure composed of hydrophilic polymeric chains that have both liquid and solid properties [10]. Like polymers, hydrogels can be either natural or synthetic. The hydrophilic nature of the polymer chains is due to the presence of any of various hydrophilic groups such as the hydroxylic(–OH), carboxylic(–COOH), sulphonic(–SO3H), or primary amidic(–CONH2) functional groups (Fariba et al., 2010). Sometimes, the hydrophilic groups are also responsible for the crosslinking of the chains, either physically or chemically, leading to the three-dimensional structure. Hydrogels are now considered a new advanced material that is promising for various applications, including but not limited to separation, medicine, food packages, agriculture, drug delivery, and water treatment [11].
The recent great interest in hydrogels is not only due to its unique characteristics and capability to absorb large amounts of water. In fact, hydrogels are very promising for separation technologies as they can selectively diffuse solutes into their pores [12]. The common synthesis components of hydrogels are the initiator, monomer, and crosslinking agent. Sometimes, more than one functional monomer is used to co-polymerise a hydrogel. Hydrogel’s absorbance and swelling behaviour is strongly influenced by the hydrophilic strength and degree of cross-linking of the polymeric chains. Other factors that may affect the hydrogel’s characteristics include the initiator’s concentration, the monomer’s concentration, reaction temperature, type of reactor, and the reaction time [13-15].
Since hydrogels are prepared in various forms including films, coatings, macro-, micro-, and nano-gels, hydrogels can be easily applied for different applications [16] . In addition, their water-absorbing properties combined with their low stiffness and highly deformable nature means that they are also widely used in biomedical devices, implants, and drug delivery systems [17].
In this research, we are investigating the fact that the rate of absorption and the amount of water absorbed are both vital characteristics of hydrogels. However, most hydrogels do not acquire both. A super-absorbent hydrogel mixture based on a high-capacity hydrogel and a high-rate absorbing hydrogel is needed, especially for water treatment. This study aims to prepare and characterize different hydrogel mixtures based on sodium polyacrylates and poly(2-acrylamido-2-methylpropane sulphonic acid) (PAMPS).
2. EXPERIMENTAL
2.1. Materials
2-Acrylamido-2-methylpropane sulfonic acid (AMPS) monomer was purchased from Alfa Aesar Company as reagent grade. It was used as received (>98% purity) without any further purification. The crosslinking agent, N,N’-methylene-bis-Acrylamide (MBA), was purchased as the analytical grade from Sigma-Aldrich Company, Germany. Ammonium persulfate (APS) initiator was purchased from Sisco Research Laboratories as reagent grade. Sodium Polyacrylates hydrogel was extracted from Molfix diapers.
2.2. Preparation of PAMPS Hydrogel
PAMPS hydrogel was prepared by free radical cross-linking solution polymerization of AMPS in water at room temperature under a nitrogen blanket in cylindrical glass tubes. The weight of AMPS added was 3.0g in 8 mL of distilled water; hence the concentration of AMPS monomer in water was 37.5wt% in all samples prepared. Different weights (0.01–1 g) of MBA crosslinker (0.33–33.33 wt%) were added. Finally, 0.01g of APS (0.33 wt%) was used as the initiator. The percentages of the initiator and crosslinker were calculated with respect to the monomer.
The AMPS monomer was dissolved in distilled water prior to mixing with MBA. Nitrogen was bubbled through the mixture at a very slow rate. Then, the APS initiator was added carefully to maintain the inert blanket. The mixture was left to cure in an oven set at 60°C for two hours.
3. RESULTS AND DISCUSSION
3.1. Effect of Crosslinker Ratio
After preparing different PAMPS samples with different crosslinking ratios ranging from 0.33% and 33.33% by weight, the water absorbed over 70 hours was measured and is plotted in Fig. (1).
Upon analysing the data, it is now evident that as the crosslinker concentration decreases, the swelling behaviour of PAMPS improves. Since different samples may not reach equilibrium at the same time, another plot was constructed taking into consideration the maximum water absorbance capacity regardless of the time factor, which is shown in Fig. (2).
It is clear that PAMPS samples with crosslinker ratio higher than 10 wt% were very rigid, which is inferred from their very poor swelling properties. While other samples with less crosslinker ratios could absorb more water. This is because as the concentration of the crosslinker is reduced, the number of crosslinks will reduce, making the distance between subsequent crosslinks longer, hence, the size of micro-pores become bigger, and eventually have room for more water molecules. These results are in agreement with Chavada’s and Patel’s work, which found that by increasing the crosslinking ratio, the porosity of the hydrogel decreases, hence the hydrogels show poorer swelling capacity [18].
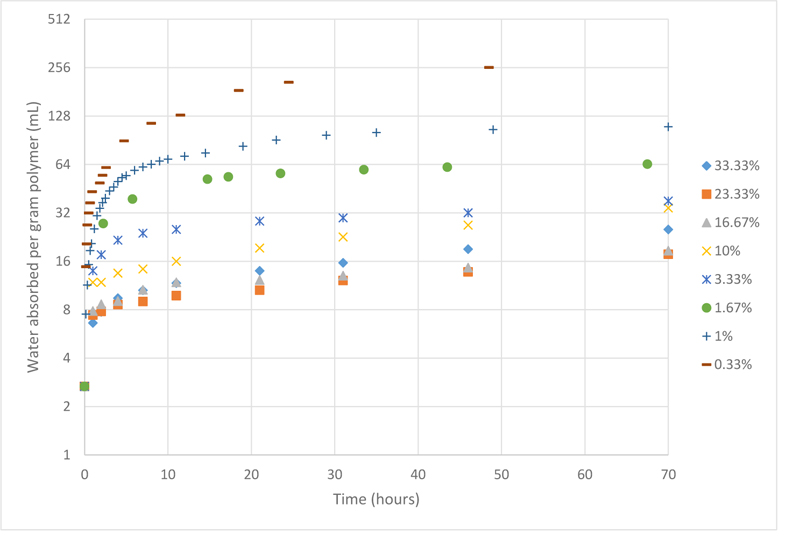
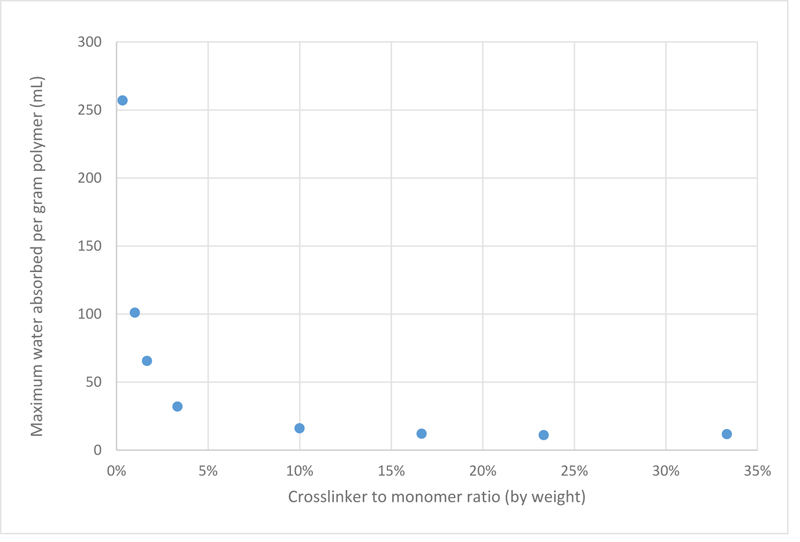
3.2. Sweeling Behaviour of PAMPS/Na-PA Mixtures
Fig. (3) shows the swelling behaviour over time of PAMPS and sodium polyacrylates (Na-PA) hydrogels. The results demonstrate how PAMPS can absorb more water at equilibrium (up to 225% its weight) compared to sodium polyacrylate (up to 150% its weight). However, Na-PA has a much faster response, reaching more than 75% of its capacity in under 5 minutes, and reaches equilibrium in a little over ten minutes. On the other hand, PAMPS takes over 15 minutes to even reach half of its capacity. This is why we seek to mix these two hydrogels; to have PAMPS’s large capacity, and Na-PA’s fast absorption.
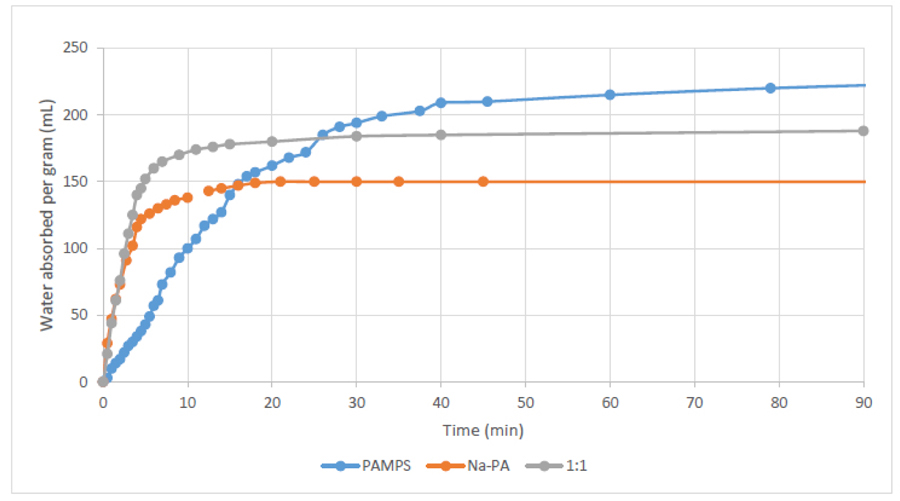
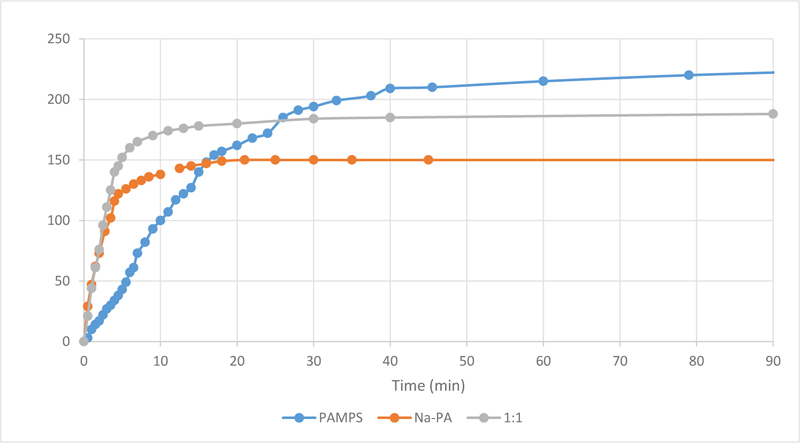
Fig. (3) also shows the swelling behaviour of a 1:1 mixture based on both hydrogels (PAMPS and Na-PA). The results prove how mixing Na-PA with PAMPS showed better swelling behaviour than using either hydrogel separately, i.e. more water absorbed than NA-PA, and higher absorption rate compared to PAMPS.
After obtaining promising results by mixing PAMPS and Na-PA, more mixtures were prepared and studied. The performance of mixtures at different ratios is presented in Fig. (4).
It is clear that increasing the PAMPS content increases the final equilibrium capacity but greatly affects the absorbance rate, and vice versa. In fact, the 1:2 mixture almost exhibits the behaviour of the pure Na-PA hydrogel, except it has a delayed response. Table 1 summarises the different swelling characteristics of each hydrogel and its mixtures. It is clear that the 1:1 mixture still shows better swelling properties and absorbance rate compared to either mixture. Therefore, the optimum mixing proportion of Na-PA and PAMPS is 1:1 as it yields a hydrogel mixture that exhibits the best swelling behaviour characterised by a fast response, high absorbance rate, and relatively large swelling capacity.
Swelling Parameter | PAMPS | Na-PA | 1:1 | 2:1 | 1:2 |
---|---|---|---|---|---|
Maximum absorbance (mL/g) | 225 | 150 | 188 | 177 | 152 |
Time to reach 50% of Eq. | 12 min | 2.5 min | 2.25 min | 3.25 min | 3.25 min |
Time to reach 75% of Eq. | 22.5 min | 3.75 min | 4 min | 8.5 min | 7 min |
Time to reach 90% of Eq. | 37.5 min | 8.5 min | 9 min | 20 min | 13 min |
Time to reach Equilibrium | 90 min | 15 min | 30 min | 85 min | 45 min |
CONCLUSION
Hydrogels are a hot topic among researchers interested in innovative and smart new materials. Hydrogels have earned this spot due to their swelling capabilities and unique characteristics. However, for new applications, a super-absorbent hydrogel that has both fast absorption rate and large swelling capacity is needed, particularly for water treatment applications. This paper investigated the swelling water behaviour of a mixture based on two hydrogels: one with large swelling capacity, and the other with very fast response.
2-Acryloylamino-2-methyl-1-propanesulfonic acid (AMPS) is an anionic vinyl monomer containing a hydrophilic sulfonic acid functional group and a non-ionic amide group. Besides, it also possesses a high tolerance towards cations. Therefore, it has been introduced into super-absorbent hydrogels to improve the performance by several works [19-30] On the other hand, sodium polyacrylate (Na-PA) is the sodium salt of polyacrylic acid. The polymer is very hydrophilic to water and is considered a super-absorbent polymer [12, 14-16]. In addition to the great swelling characteristics, Na-PA has several mechanical advantages, including its stability and thermal resistance. This has led to the use of Na-PA in a wide range of applications [29-34]
This paper investigates the effect of crosslinker ratio on the swelling capacity of PAMPS. It was found that as the crosslinking ratio decreases, the porosity of the hydrogel increases, thus improving the swelling capacity. These results are in agreement with the literature [8]. This paper also investigated the swelling behaviour of different hydrogel mixtures based on PAMPS and Na-PA. The results showed that a 1:1 mixture has better swelling properties compared to each hydrogel separately in terms of swelling capacity and rate of absorption.
CONSENT FOR PUBLICATION
Not applicable.
AVAILABILITY OF DATA AND MATERIALS
The source of data and materials are archived in the BUE "British University in Egypt" library.
FUNDING
This research was funded by the BUE "British University in Egypt" under grant number NTRC-FAS2019-01.
CONFLICT OF INTEREST
Dr. Elazab A. Hany is the Editorial Advisory Board Member of The Open Chemical Engineering Journal.
ACKNOWLEDGEMENTS
Declared none.