All published articles of this journal are available on ScienceDirect.
Mathematical Modeling of Sorptive Extraction of Lithium Chloride from Lithium-containing Brine of the Aral Sea Region
Abstract
Introduction
The article presents the results of chemical and physicochemical analysis of initial lithium-containing hydromineral raw materials and sorbent based on bentonite clay with titanium oxide addition.
Methods
It is established that the mineral is montmorillonite group and kaolinite according to the results of the analysis of the microstructure and elemental composition of bentonitic clay of the Darbazinsky deposit. Brine is characterized by the change of lithium chloride content in the range of 403-1001 mg/l. Physical and mechanical characteristics of the obtained sorbents based on bentonite clay and titanium oxide additive are characterized by a high mechanical strength of 5.31 MPa, density of up to 2,6 g/cm3, and specific surface area of 1572 cm2/g. The technological parameters of sorption extraction of lithium chloride from lithium-containing hydromineral raw materials were optimized using the system analysis “STATISTICA” developed by StatSoft company. It is established that to increase the rate of lithium chloride extraction from brine, it is necessary to maintain the rate of brine flow within 8 l/min at maximum humidity of solution output up to 43g/m3 on the basis of the obtained volumetric graphical dependencies.
Results
The microstructure of the used sorbents with titanium oxide addition up to 20% is characterized by the predominance of lithium chlorides, which are represented by heap-shaped, prismatic crystals.
Conclusion
The maximum amount of lithium chloride in the form of clusters of light-white crystals of tabular shape on the surface of the sorbent is observed at increasing the content of titanium oxide up to 40%.
1. INTRODUCTION
Lithium is the lightest metal with a number of unique properties. In this regard, both metallic lithium and its various compounds are widely used in various fields of production in highly developed countries of the world. The most capacious areas of use of lithium products are aluminum electrolysis and glass and ceramics production [1]. In addition, lithium and its compounds are used in the production of lubricants [2], synthetic rubber [3], electric batteries [4], and other fields of technology [5]. However, limited reserves of solid lithium ores and high costs associated with its extraction indicate the efficiency of lithium extraction from brines using promising techno- logies [6].
Natural-rich lithium ores and brines are located mainly in deposits in Chile, while in other countries, the lithium content in both ores and brines is much lower. Therefore, the separation of lithium compounds is labor-intensive and multi-stage. There are huge reserves of lithium-containing brines in Bolivia, Argentina, China, Russia, and Kaza- khstan, as well ass Chile. The low competitiveness of the existing mineral resource base and difficult conditions for the development of traditional types of processing of lithium-containing raw materials, which require in-demand production of lithium products, is an urgent problem.
This research aims to study the physicochemical features of lithium-containing brines and optimize the process of sorption extraction of lithium chloride.
Lithium is found in mineral resources containing spodumene (LiAlSi2O6) and hectorite (Na0.3 (Mg, Li)3Si4 O10(OH)2 minerals [7]. Extraction of lithium from mineral rocks is difficult and requires significant economic costs due to the content of impurity and associated metals the difficulty of access to mineral veins [8]. Lithium is also found in the brines of desert basin lakes, silt of saline lakes, and seas, with variously variable concentrations of about 200-4000 mg/l Li+, which makes exploration and resource estimation expensive. However, the extraction of lithium from natural brines is less costly than the extraction of lithium from solid mineral rocks.
The sedimentary and mud compositions of the Northern Aral Sea contain large reserves of lithium compounds in a mixture of chloride and sulfate salts of alkaline and alkaline-earth metals in Kazakhstan. The brines of the Aral Sea and the silt of the basin and Lake Zhaksykylysh are characterized by significant fluctuations in lithium chloride content of 120-360 mg/kg, while in the silt, the content reaches up to 1050 mg/kg [9, 10]. Lithium-containing hydro-mineral resources of Kazakhstan are a promising material for obtaining lithium compounds in demand in the form of chloride, carbonate, and others.
Lithium is isolated from brines by adding sodium carbonate (Na2CO3) with precipitation of Li+ in the form of Li2CO3. However, extraction processes are used to obtain high-purity Li2CO3 [11]. Adsorbents in various forms of compounds were previously used [12], such as magnesium oxide [13], titanium dioxide [14], and lithium-aluminum hydroxides [15] in the process of obtaining lithium salts from hydromineral raw materials. An analysis of modern lithium extraction methods has shown the advantages of selective sorption isolation of lithium chloride using various aluminate sorbents [16].
Bentonite clays are widely used as adsorbents for the isolation of various substances, including metals Cr3+, Pb2+, and Cd2+ [17], and Eu (III) [18]. The presence of cations on the crystal surface strongly affects its adsorption activity, although its mechanism is still unclear [19]. Alumino- silicate adsorbents are non-porous and require activation and heat treatment to give mechanical strength.
The authors investigated the sorption isolation of alkali metal chloride from natural marine waters and lake brine using the H2TiO3 sorbent and allowed the isolation of Li+ with a concentration of 98.86% [20]. It is important to take into account the economic component of the raw materials for the selection of the adsorbent when studying the adsorption process. In this regard, the development of a sorbent based on local bentonite clays for the sorption isolation of lithium chloride from the brine of the Aral Sea of the Zhaksykylysh deposit will reduce the cost of final products.
The addition of titanium dioxide will help to increase the strength of the aluminosilicate sorbent and ensure the desorption of lithium chloride. A sorbent based on bentonite clay with the addition of titanium dioxide is planned to be used to conduct research on the sorption isolation of lithium chloride from the brine of the Aral Sea.
2. MATERIALS AND METHODS
2.1. Materials
Hydroaluminosilicate Bentonite clay Al2[Si4O10](ОH)2 ·nH2О was used as an adsorbent (Darbazinskoye field in the southern region of the Republic of Kazakhstan), after preliminary grinding to 1-2 microns.
TiO2 titanium dioxide was used as an additive to increase the sorption separation of lithium chloride (Titanium Investments LLC, Russian Federation).
Sulfuric acid (concentration 68%) was used for activation by the plant of mineral fertilizers Kazphosphate LLP (Taraz, Kazakhstan).
Acid activation of bentonite was carried out with sulfuric acid with a concentration of 0.2M, 0.6M, and 2.0M to prepare the sorbent. Activation was performed for 24 hours at room temperature. After activation was completed, the suspension was filtered with a vacuum filter and rinsed with water until complete purification from the acid residue. The resulting wet mass was mixed with a predetermined amount of titanium dioxide for subsequent granulation.
Granulation was carried out on a plate granulator to the size of granules of 5-8 mm. The obtained granules were dried at a temperature of 100°C in a drying cabinet for 1 hour. The dried granules were sent for heat treatment.
The heat treatment was carried out in a laboratory muffle furnace (SNOL-1300, Russian Federation) at a temperature of 800°C with a duration of 60 minutes to increase the mechanical strength and porosity of the sorbent.
The laboratory adsorption unit with software control of the technical and technological parameters of MeasLAB was used for the sorption isolation of lithium chloride from the studied brine (Fig. 1).
The laboratory adsorption unit operated according to the following scheme. The lithium-containing brine came from compressor 1 through a flow meter with needle valve 2 and was sent to flask 3, in which it was heated to a predetermined temperature. The moisture content of the air was measured by a relative humidity sensor 7. The humidity and air temperature parameters are displayed on digital TRM200 devices (6). The water temperature was adjusted by the TC4S system (9). The maximum permissible water temperature is 70°C. The prepared sorbents were loaded into flask 4, and the lithium-containing brine under study was pumped through flask 4 for sorption at a predetermined feed rate after heating.
2.1.1. Mathematical Processing of Experimental Adsorption Data
Mathematical processing of experimental adsorption data was carried out according to the program using the N.A. Shilov equation:
![]() |
(1) |
where τ - the time of the protective action of the layer, sec;
К - sorption action coefficient, s/m, showing the time of sorption action of a layer with a length of 1 m;
L - layer length, m;
τ0- loss of sorption action time.
The sorption output curve is usually measured when studying the sorption process - the change in the concentration of the absorbed substance overtime behind the adsorbent layer. According to the detailed equation of sorption dynamics:
![]() |
(2) |
The volumetric velocity of the vapor-gas mixture W was determined by the flow equation:
![]() |
(3) |
The moisture content of the air at the inlet x0 and at the outlet x of the adsorption column for each moment of time was determined by the formula:
![]() |
(4) |
where Рс - the pressure of saturated water vapor in the air at a dry temperature of tc thermometers, mmHg.
The pressure of saturated water vapor was calculated using Eq. (5), which for water vapor in the temperature range from 0 to 60°C has the form:
![]() |
(5) |
![]() |
(6) |
2.2. Methods of Analysis
The flame photometric method was used to analyze lithium-containing brine by its metallic component. The flame atomic absorption spectrometer contrAA 300 (AnalytikJena AG, Germany) is a new generation spectrometer with a continuous-spectrum xenon lamp for analysis of all elements. Each analyzed element does not require separate hollow cathode lamps. It requires high analysis accuracy and reproducibility.
Studies of the microstructure of adsorbent samples before and after adsorption were carried out on a JEOL JSM 6490 LV scanning low vacuum electron microscope (Japan) with INCA Epegdu 350 energy dispersive microanalysis systems (Oxford Instruments, UK) and HKL Basic polycrystalline sample texture analysis system (Oxford Instruments, UK).
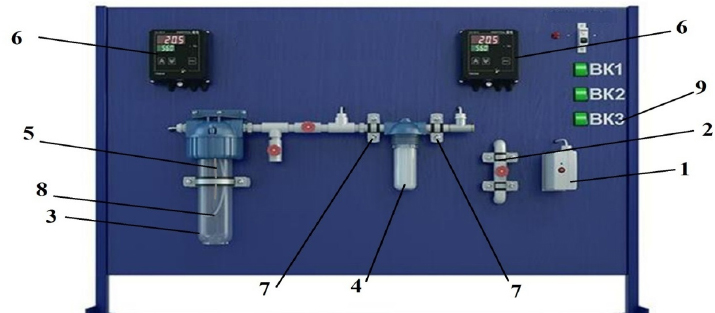
The scheme of the adsorber installation.
1 – compressor, 2 – flow meter, 3 – flask with the studied brine, 4 – flask with an adsorber, 5 – ten, 6 – digital TRM converter, 7 – humidity sensors, 8 – air divider, 9 – TC4S heating regulator.
The physico-mechanical strength of the sorbent was analyzed on an ultrasonic hardness tester TKM-459 (Russia).
The initial raw material of bentonite was mixed with water, granulated after pre-drying, and subjected to heat treatment in a muffle furnace at a temperature of 800°C and a duration of 60 minutes to study the mechanical strength of bentonite granules. After cooling, the heat-treated granules were tested for mechanical strength on a hardness tester.
Acid activation of these compounds was carried out after studying the composition and physico-mechanical features of the sorbent based on aluminosilicate materials and titanium compounds.
Acid activation of sorbent samples based on aluminosilicate materials of titanium and lithium chloride compounds was carried out with sulfuric acid 0.2M, 0.6M, and 2.0M.
Acid–activated sorbent samples were pre-dried to a mass-like state after washing. The mixed compositions were granulated and heat treated at temperatures of 700-1000°C with an isothermal exposure of 60 minutes. Changes in the physico–mechanical parameters of heat-treated granules at a temperature of 1000°C and a duration of 60 minutes were reported.
The specific surface area of dispersed materials was determined by the PSX-K device (Russia). It allows measuring the specific surface area in the range of 100-50000 cm2/g. The average mass size of powder particles is 0.1–250 microns with an average measurement error of ± 1.5%.
The degree of lithium chloride release was calculated as the ratio of the lithium salt content in the initial brine to the lithium salt content in the final solution after adsorption.
Mathematical modeling of the sorption process was carried out using the STATISTICA program (version 10.0) developed by StatSoft [21]. The technological parameters of the process, such as temperature, flow rate, and inlet and outlet humidity, are determined based on the volumetric graphical dependences of lithium chloride release obtained under experimental conditions.
3. RESULTS AND DISCUSSION
An analysis of modern lithium extraction methods has shown the advantages of selective sorption isolation of lithium chloride since it allows for the maximum degree of lithium extraction from aqueous solutions [22].
The presence of cations on the surface of bentonite crystals strongly affects its adsorption activity. Positive one-, two- and trivalent cations are located on the surface of the package due to the negative charge of the hydro- aluminosilicate anions. These are mainly Na, K, Ca, Mg, and Fe. Hydrate shells can form around these cations, and the aggregate of packages swells as a result of interaction with water. It is characteristic that the volume of the hydrate shell varies for different cations. Alkali metal ions and, first of all, sodium have the greatest hydrating ability. Ions of alkaline earth metals, such as calcium and magnesium, have a significantly lower hydrating ability, which negatively affects the adsorption properties. Calcium and magnesium are eliminated by acid activation, washing them off in sulfate form [23, 24]. The chemical composition of bentonite clay of the Darbazinsky deposit is demonstrated in Table 1.
The results of the element composition and the electronic image of the bentonite clay microstructure are demonstrated in Fig. (2).
There is a significant predominance of minerals in the montmorillonite group of scaly aggregates, and the SiO2:R2O3 ratio reaches 6-8 in the microstructure of the bentonite clay sample of the Darbazinsky deposit. The hydrosludes in bentonites are represented by minerals of hydrargyllite in the form of hexagonal benzene plates and kaolinite in the form of pseudohexagonal scales in the form of irregular plates and worm-like aggregates.
The test results of the physical and mechanical analysis of sorbents are shown in Table 2.
The results of mechanical strength studies have shown that bentonite clay granules have up to 3.0 MPa. These results indicate the suitability of this bentonite clay as a sorbent.
The Content of the Components, % | ||||||||
---|---|---|---|---|---|---|---|---|
СаО | Fe2O3 | MgO | SiO2 | Al2O3 | TiO2 | Na2O + К2O |
P2O5 | S |
8.49 | 5.2 | 3.03 | 42.30 | 20.55 | 1.95 | 2.84 | 0.12 | 0.36 |
The Composition of the Mixtures, % | Weight before Firing, g | Weight after Firing, g | Firing Conditionsа | The Diameter of the Granules, mm | Mechanical Strength of Granules, MPa |
|||
---|---|---|---|---|---|---|---|---|
Bentonite, g |
Water, ml | Т,°С | t, min | Before Firing | After Firing | |||
200 | 80 | 239 | 186.35 | 800 | 60 | 3-3.2 | 4.8-5 | 3.2 |
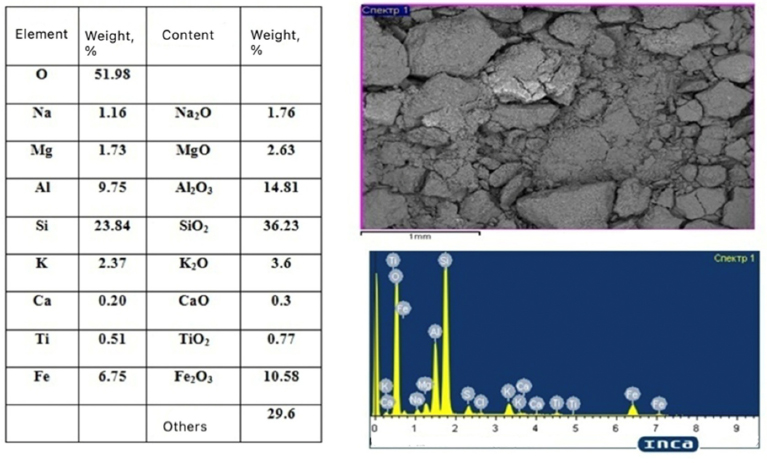
Microstructure and element composition of bentonite clay.
The results of the chemical analysis showed that the content of acidic components such as SiO2 and Al2O3 increases as the molarity of sulfuric acid increases. The content of alkaline and basic oxides, as well as titanium and iron oxides, decreases. These changes seem to be associated with their transition to sulfate solutions, which are washed off as sulfate compounds during washing (Table 3).
The results of changes in the physico–mechanical parameters of heat-treated granules at a temperature of 1000 ° C and a duration of 60 minutes are demonstratedin Table 4. Analysis of the data obtained showed that the physico-mechanical characteristics improve with the addition of up to 30% titanium oxide to the bentonite composition. At the same time, the mechanical strength reaches 5.31 MPa, the average diameter of the pellet is 18.9 mm, and the specific surface area is 1560 cm2/g.
The results of the analysis of the compositions of brines and silt of salt lakes in the Aral Sea region are presented in Tables 5 and 6 to conduct experiments using the proposed technology [25].
The Content of the Components | Inactive Sorbent | Activated with Sulfuric Acid | ||
---|---|---|---|---|
0.2 М | 0.6 М | 2.0 М | ||
- | - | - | Content | - |
SiO2 | 53.61 | 50.2 | 51.3 | 52.3 |
Al2O3 | 11.22 | 11.7 | 11.9 | 12 |
Fe2O3 | 5.6 | 5.6 | 5.3 | 4.8 |
TiO2 | 1.2 | 1.2 | 1.0 | 0.8 |
Na2O | 0.63 | 0.4 | 0.33 | 0.2 |
K2O | 1.4 | 1.3 | 1.2 | 1.0 |
CaO | 2.9 | 2.2 | 1.7 | 1.1 |
MgО | 1.0 | 0.9 | 0.6 | 0.5 |
Sorbent Composition | Density, g /cm3 | Specific Surface Area, cm2/g | Average Diameter of Pellets, mm | Strength, MPa |
---|---|---|---|---|
Acid-activated bentonite | 1.93 | 1396 | 15.9 | 4.8 |
Acid-activated bentonite with the addition of 10% titanium oxide | 2.29 | 1420 | 17.9 | 5.11 |
Acid-activated bentonite with the addition of 15% titanium oxide | 2.44 | 1511 | 18.3 | 5.23 |
Acid-activated bentonite with the addition of 20% titanium oxide | 2.56 | 1559 | 18.3 | 5.29 |
Acid-activated bentonite with the addition of 25% titanium oxide | 2.6 | 1572 | 18.3 | 5.31 |
Sample Number | 1 | 2 | 3 | 4 |
---|---|---|---|---|
Li | 264 | 312 | 128 | 142 |
B | 103 | 751 | 95 | 226 |
Na | 70640 | 89000 | 76000 | 83490 |
Mg | 23510 | 43707 | 17750 | 19030 |
Al | 25 | 237 | 17 | 10 |
La | 0.005 | 0.021 | 0.005 | 0.0025 |
Ce | 0.008 | 0.021 | 0.005 | 0.01 |
Pr | 0.003 | 0.005 | 0 | 0 |
Sample Number | 1 | 2 | 3 | 4 |
---|---|---|---|---|
Li, mg/kg | 1002 | 1051 | 415 | 403 |
The Content of the Main Component m, mg/l | Dynamic Capacity, meq/g | Degree of Sorption α,% | V, min |
---|---|---|---|
200 | 140 | 54.5 | 0.25 |
300 | 160 | 57.2 | 0.20 |
400 | 180 | 59.01 | 0.18 |
500 | 246 | 68.7 | 0.17 |
600 | 220 | 77.8 | 0.23 |
700 | 237 | 79.5 | 0.26 |
800 | 242 | 82.3 | 0.24 |
900 | 246 | 86.4 | 0.23 |
1000 | 252 | 90.5 | 0.25 |
It can be seen that the lithium content in brine ranges from 128 to 264 mg /kg, and the content of rare earth elements is insignificant from the data in Table 5. The content of Na and Mg is many times higher than that of lithium chloride. Sodium 70640 to 89000 mg/kg. Magnesium 17750 to 43707 mg/kg.
It can be seen that the lithium content in brine varies in grades from 128 to 263 mg/kg and in silts from 403 to 1051 mg/kg from the data in Table 6. Lithium extraction from such raw materials is possible by sorption or extraction from brines using an effective reagent.
The dependence of the degree of lithium chloride release from the brine on the dynamic capacity and linear filtration rate is shown in Table 7.
It follows from the data in Table 7 that the maximum degree of lithium chloride release in the sorbent is observed at m = 1000 mg/l and is 90.5% in accordance with the dynamic capacity of the sorbent 252 and the filtration rate of 0.25 minutes.
Comparative results of experimental and theoretical data showed slight deviations in the data of the degree of sorption (α) at a process duration of 35-50 seconds (Fig. 3). The analysis of the obtained graphical dependencies showed that an increase in temperature, the flow rate of the liquid under study, and the duration of sorption contributes to an increase in the degree of lithium chloride release on the sorbent.
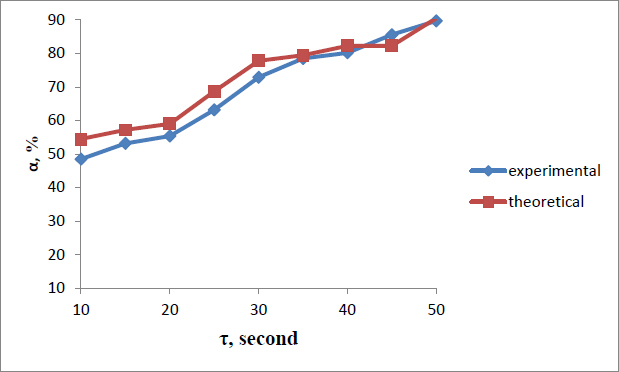
Dependence of the degree of isolation of lithium chloride from brine.
Further prolongation of the duration does not significantly affect the degree of lithium chloride release.
The so-called “star shoulder” (shoulder of star points) is used to build rotatable central compositional plans. The value of the “star shoulder” is φ=1.414 (on a coded scale) for two factors. The temperature and duration of the process were independent factors.
As a result of the mathematical processing of the experimental results, the coefficients b of the regression equation describing the object under study were determined in the form:
αLi = b 0 + b1.x1 + b2.x2 + b11.x12 + b22.x22 + b12.x1x2,
where αLi - the degree of lithium release.
The significance of the coefficients of the equation was checked according to the Student's criterion. The use of the Fisher criterion confirmed the adequacy of the obtained equation.
In encoded form, the regression equation has the form:
αLi = 85,06 + 2,45.x1 + 0.x2 + 2,55.x3 + 1,87.x12 + 1,81.x22 + 0.x32 + 0.x1.x2 + 2,94.x2.x3
which, after processing the independent coefficients according to the Student's criterion, takes the form:
αLi = 85,06 + 2,45.x1 + 2,55.x3 + 1,87.x12 + 1,81.x22 + 2,94.x2.x2
Based on the obtained volumetric graphical dependences of lithium chloride release under experimental conditions, the technological parameters of the process, such as temperature, flow rate, inlet, and outlet humidity, are determined.
The analysis of three-dimensional graphical dependences of the influence of temperature, brine consumption, sorption duration, inlet and outlet humidity on the degree of sorption isolation of lithium chloride is presented in Figs. (4-6).
As follows, from the analysis results obtained, the degree of sorption of lithium chloride is significantly affected by the humidity of the inlet and outlet, as well as the brine consumption (Fig. 4). At the same time, a maximum increase in the moisture content of the solution output to 43 g/m3 is observed, with an increase in the brine consumption to 8 l/min. The maximum release of lithium chloride on the sorbent is achieved by 90% with a process duration of 70 seconds (Figs. 5, 6).
The analysis of the obtained microphotographs after sorption isolation of lithium chloride is shown in Fig. (7).
Micrography and crystal structure when using sorbents with a 20% addition of titanium oxide is characterized by a predominance of sodium chlorides, which are represented by cumulus, prismatic crystals (a). When using sorbents with an addition of 30% to 40% titanium oxide based on bentonites, the maximum amount of lithium chloride is observed in the form of clusters of light white tabular crystals (b, c).
Thus, the isolation of lithium chloride by the sorption method using sorbents based on local bentonite clays with the addition of titanium oxide makes it possible to increase the degree of isolation of lithium chloride.
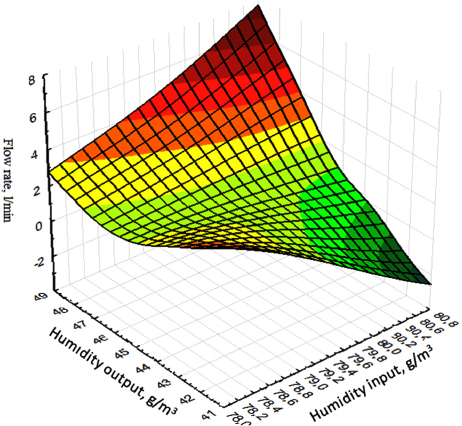
Dependence of inlet and outlet humidity on brine consumption.
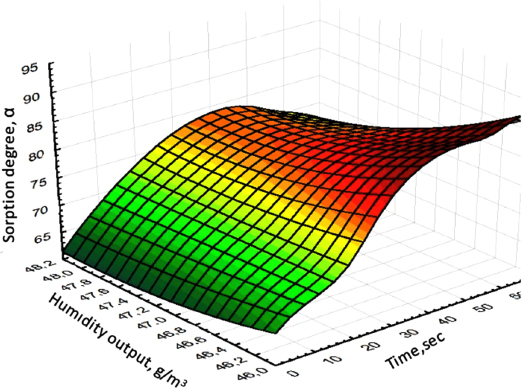
Dependence of the degree of sorption of lithium chloride on the time and humidity of the output.
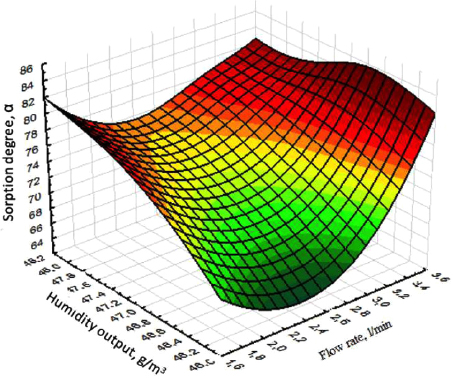
Dependence of the degree of sorption of lithium chloride on the time and humidity of the inlet.
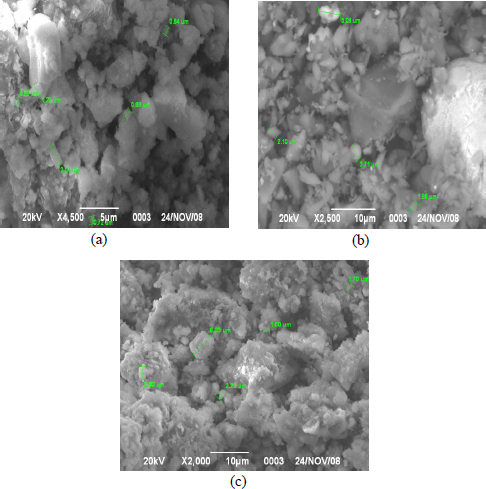
(a-c) Micrographs of sorbents after sorption isolation of lithium chloride from hydromineral raw materials.
CONCLUSION
The analysis of the data presented in the article on the spread of lithium-containing mineral resources and the widespread growth in demand for its compounds requires the development of rational methods for extracting this metal. The physicochemical methods of analysis of lithium-containing hydromineral resources of the Aral Sea region have established the features of lithium compounds in the form of impurities of chloride and sulfate salts of alkaline and alkaline earth metals.
The sorbent composition was selected, including local bentonite clay from the Darbazinsky deposit and titanium oxide, to ensure high mechanical strength for maximum sorption separation of lithium chloride from brines of the Aral Sea region. The sorption activity of bentonite clay increases with sulfuric acid activation at a concentration of 68%. At the same time, the maximum changes in the concentrations of alkaline earth metals, calcium, and magnesium reach 50%.
The dependence of the degree of lithium chloride separation on the dynamic capacity of the sorbent and the linear filtration rate was established as a result of experimental studies of the sorption separation of lithium chloride from brines, depending on the content of the useful component. The maximum degree of lithium chloride release on the sorbent is achieved with a dynamic capacity of 252 meq/g and a lithium chloride content in the brine of 1000 mg /l, with a linear velocity of 0.25 minutes.
Three-dimensional dependences of the degree of sorption of lithium chloride on the proposed sorbent showed the possibility of maximum release up to 90% with a decrease in humidity to 46% at the outlet and a duration of 70 s.
AUTHORS’ CONTRIBUTION
M.U. and D.P contributed to the study’s concept and design, S.T. wrote the paper, and A. and A.T. analyzed the data and interpreted. All authors reviewed the results and approved the final version of the manuscript.