All published articles of this journal are available on ScienceDirect.
KCo1/2Hf1/2(MoO4)2 – A Novel Glaserite-like Molybdate Exhibiting Two-dimensional Negative Thermal Expansion
Abstract
Introduction
Due to the peculiarities of the crystal structure and composition, complex molybdates exhibit valuable functional properties. Although the intensive search for NTE materials has been going on for many years, the investigation of the mechanisms and structure-property relationships causing the “thermal shrinkage” effect is still relevant. Here, we report on the 2D-NTE effect in a new ternary molybdate KCo1/2Hf1/2(MoO4)2 with a layered glaserite-like structure, and its thermal deformations and ionic conductivity were investigated.
Methods
The KCo1/2Hf1/2(MoO4)2 was synthesized through the ceramic method. HT-PXRD patterns were acquired using a Bruker D8 ADVANCE diffractometer and subsequently utilized for Rietveld analysis. Structure refinement and lattice parameter calculations were conducted with TOPAS 4.2, while calculation of the thermal expansion tensor and visualization were carried out in the TTT. ATR-IR spectroscopy was used to characterize the coordination of the molybdenum atoms. TG and DSC analyses were performed using an STA 449 F1 Jupiter thermal analyzer. Electrical conductivity was assessed using a Z-1500J impedance meter using the two-contact impedance spectroscopy method.
Results
KCo1/2Hf1/2(MoO4)2 crystallizes with the trigonal glaserite-type structure in space group P3-m1 with unit cell parameters of a = 5.76190 (3), c = 7.14788 (5) Å, V = 205.51 (1) Å3, and Z = 1. DSC indicated that KCo1/2Hf1/2(MoO4)2 melted at 699°C with decomposition. The observed 2D negative thermal expansion in the ab plane did not lead to a reduction in the cell volume. The studied ternary molybdate KCo1/2Hf1/2(MoO4)2 (αV = 148×10–6 °С–1 at 600°С) can be categorized as a high-expansion material.
Conclusion
KCo1/2Hf1/2(MoO4)2 has been categorized as a high-expansion material (αV = 148 × 10− 6 ◦С− 1 at 600◦С). At higher temperatures, the compound demonstrated notable ionic conductivity, values up to 0.42·10–3 S/cm (570°C) at Еа = 0.8 eV.
1. INTRODUCTION
Due to the peculiarities of the crystal structure and composition, complex molybdates and tungstates exhibit valuable functional properties, including ion-conducting [1-4], magnetic [5], ferroelectric [6], photocatalytic [7], laser [8], luminescent [9-12] and others [13-15]. Compounds with negative thermal expansion (NTE), which have the capability to contract along specific directions with increasing temperature, also attract much attention. They are used to compensate for the total thermal expansion of functional materials operating under extreme conditions. Representatives of well-known structural families with different mechanisms are used as NTE materials [16-20]. It is known that compounds with a layered structure have high structural anisotropy and, in some cases, can exhibit two-dimensional (2D) NTE behavior [21-24]. Although the intensive search for NTE materials has been going on for many years, the investigation of the mechanisms and structure-property relationships causing the “thermal shrinkage” effect is still relevant [25-27]. Here, we report on the 2D-NTE effect in a new ternary molybdate KCo1/2Hf1/2(MoO4)2 with a layered glaserite-like structure, and its thermal deformations and ionic conductivity were investigated.
2. METHOD
2.1. Sample Preparation
The glaserite-like ternary molybdate KCo1/2Hf1/2(MoO4)2 was synthesized through the ceramic method using stoichiometric quantities of K2MoO4 (chemically pure, Krasny Khimik Ltd., Russia) and pre-synthesized molybdates CoMoO4, Hf(MoO4)2. Stepwise annealing of the simple molybdate mixture was carried out in a muffle furnace at 450–580 °C for 150 h. Hafnium molybdate Hf(MoO4)2 was prepared from a stoichiometric mixture of HfO2 (chemically pure, IGIC RAS, Russia) and MoO3 heated at 350-750°С for 100 h. The simple molybdate CoMoO4 was synthesized using a stoichiometric mixture of CoO (chemically pure, Krasny Khimik Ltd., Russia) and MoO3, subjected to heating at 350–600 °C for 100 hours. The initial reagents were thoroughly mixed and ground in an agate mortar. Following each heating cycle, the samples were ground again and analyzed using XRD.
2.2. Variable Temperature Powder X-ray Diffraction
X-ray powder diffraction data for KCo1/2Hf1/2(MoO4)2 were acquired at room temperature using a Bruker D8 ADVANCE diffractometer (VANTEC detector, CuKα radiation) and subsequently utilized for Rietveld analysis. Scanning was performed over a range of 5 and 100° with a step of 0.02° and a speed of 0.1 s−1. High-temperature powder X-ray diffraction (HT-PXRD) measurements were conducted in an Anton Paar HTK16 high-temperature chamber over a range of 30–600 °С with 50 °С increments. Structure refinement and lattice parameter calculations were conducted with the TOPAS 4.2 software [28], while calculations of the thermal expansion tensor and visualization were carried out in the TTT software package [29].
2.3. ATR-IR Spectrum
Attenuated total reflectance infrared spectroscopy (ATR-IR) was used to characterize the coordination of the molybdenum atoms. ATR-IR spectra were acquired using a SIMEX FT-801 FT-IR spectrometer equipped with a diamond ATR cell at room temperature within the range 500–1100 cm−1 with a resolution of 1 cm−1.
2.4. Thermal Analysis
Thermogravimetric (TG) and differential scanning calorimetric (DSC) analyses were conducted using an STA 449 F1 Jupiter thermal analyzer (NETZSCH). Thermal analysis was performed in platinum crucibles across a temperature range of 30–750°C under an argon atmosphere, with a heating rate set to 10°C/min. The temperature was measured with an accuracy of ±1° using a Pt-PtRh thermocouple. Before the experiments, the blank measurement was made with an empty crucible to register the drift correction.
2.5. Impedance Spectroscopy
To study ion transport in KCo1/2Hf1/2(MoO4)2, its ceramic sample in the form of disk-shaped pellets (d=10 mm, h=1.9 mm) was used. Ceramic disks for electrophysical studies were prepared by pressing powder at a pressure of 1 kbar. The platinum electrodes were deposited on the flat surfaces of the pellets. For this purpose, a method of precipitation of colloidal platinum on large surfaces of tablets was used, followed by annealing for an hour at a temperature of about 600°C. Electrical conductivity was assessed using a Z-1500J impedance meter over a temperature range of 100–570°С using the two-contact impedance spectroscopy method in both heating and cooling modes (2°C/min, frequency range of 1–106 Hz).
3. RESULTS AND DISCUSSIONS
3.1. Rietveld Refinement
The refined lattice parameters for obtained KCo1/2Hf1/2(MoO4)2 are shown in Table 1, main interatomic distances – in Table 2, the atomic coordinates and isotropic displacement parameters – in Table 3. The data are in good agreement with those published earlier for KMg1/2Zr1/2(MoO4)2 [30]. The parameters and, accordingly, the volumes of the unit cells decrease consistently (see Table 1).
(Fig. 1) presents the calculated and experimental XRD curves, along with the corresponding difference curve.
Compound | KCo1/2Hf1/2(MoO4)2 | KMg1/2Zr1/2(MoO4)2/Refs. |
---|---|---|
Sp.gr. | Trigonal, P3-m1 | |
R(M2+) Å, C.N. = 6 | 0.79 [31] | 0.86 [31] |
a, Å | 5.76190 (3) | 5.7679 (1) [30] |
c, Å | 7.14788 (5) | 7.1744 (1) [30] |
V, Å3 | 205.51 (1) | 206.70 (1) [30] |
Z | 1 | 1 |
2θ-interval, ° | 5–100 | |
Rwp, % | 2.901 | |
Rp, % | 2.134 | |
Rexp, % | 1.134 | |
χ2 | 2.559 | |
RB, % | 1.991 |
MoO4 – tetrahedron | (Co/Hf)O6 – octahedron | ||
---|---|---|---|
Bond | d | Bond | d |
Mo—O(1) | 1.684 (7) | (Co/Hf)—O(2) | 2.138 (4) х6 |
Mo—O(2) | 1.691 (5) х3 | ||
(Mo—O) | 1.689 | ||
KO12 – icosahedron | |||
K—O(1) | 3.3277 (2) х6 | K—O(2) | 2.881 (4) х6 |
Atom | x | y | z | Occ. | Biso |
---|---|---|---|---|---|
Hf | 0 | 0 | 0 | 0.5 | 0.86 (10) |
Co | 0 | 0 | 0 | 0.5 | 0.86 (10) |
K | 0 | 0 | 1/2 | 1 | 1.19 (15) |
Mo | 1/3 | 2/3 | 0.2524 (3) | 1 | 0.72 (6) |
O1 | 1/3 | 2/3 | 0.488 (1) | 1 | 0.84 (13) |
O2 | 0.1727 (4) | 0.3455 (9) | 0.1770 (5) | 1 | 0.84 (13) |
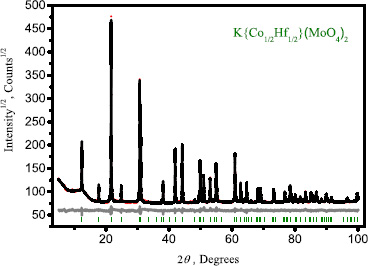
Difference Rietveld plot of KCo1/2Hf1/2(MoO4)2.
We used fractional coordinates of K(Mg0.5Zr0.5)(MoO4)2 in our initial structural model () [32]. The peak shapes were described using the Pearson VII function with a correction for sample texture along the 001 direction applied using the March-Dollase function.
The refinement process involved the stepwise introduction of refinable parameters, with simultaneous graphical modeling of the background.
The final refinement demonstrated stability, yielding low residual R factors. The crystallographic data for KCo1/2Hf1/2(MoO4)2 were deposited with the Cambridge Crystallographic Data Centre (CSD 2393645). The data are accessible on the CCDC website (www.ccdc.cam.ac.uk/ data_request/cif).
The KCo1/2Hf1/2(MoO4)2 structure exhibits a layered crystal structure. Two distinct layers are oriented perpendicular to the c-axis: the first layer comprises (Co/Hf)O6 octahedra, while the second consists of KO12–icosahedra and MoO4–tetrahedra, which are connected through shared oxygen edges (Fig. 2).
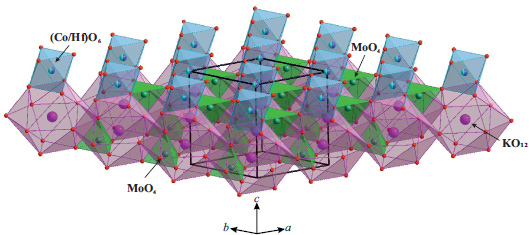
The crystal structure of KCo1/2Hf1/2(MoO4)2.
3.2. ATR-IR Spectrum
The IR spectra of complex molybdates contain bands characteristic of vibrations of the tetrahedral MoO4-groups. Usually, bands corresponding to stretching (980–700 cm-1) and bending modes (410–300 cm-1) of Mo–O bonds are observed [33]. The experimental IR spectra encompass only the stretching vibrational modes of the MoO4 tetrahedra. The IR spectrum of the compound (ν1 = 899 cm-1; 862 cm-1; 829cm-1; ν3 = 727cm-1) confirmed the presence of MoO4−tetrahedra and exhibited a strong similarity to the spectrum of the isostructural compound KMg1/2Zr1/2(MoO4)2 [30] with isolated MoO4 tetrahedra (Fig. 3).
3.3. Thermal Properties
The DSC curves recorded from KCo1/2Hf1/2(MoO4)2 are shown in (Fig. 4). Upon heating the sample, two endothermic signals were observed at 490°С and 699°С. The endothermic peak at 699°С indicated the melting point of KCo1/2Hf1/2(MoO4)2. No weight loss was observed on the TG curve.
The Rietveld-refined XRD patterns of KCo1/2Hf1/2(MoO4)2 after annealing at 750°С indicated its partial decomposition, leading to the formation of CoMoO4, K2Mo3O10, K2Co2(MoO4)3, and HfO2.
To further investigate the endothermic effect observed at 490°С, the sample was analyzed in a “heating-cooling” mode within the temperature range of 200–650°С. During cooling, KCo1/2Hf1/2(MoO4)2 exhibited an exothermic effect at 473°С, with a hysteresis of 17°С. The characteristic signal within the 473–490°С range indicated the presence of a reversible phase transition (type I) in KCo1/2Hf1/2(MoO4)2 (Fig. 4).
The temperature dependences of the lattice parameters of KCo1/2Hf1/2(MoO4)2, resulting from the HT-PXRD experiments, are presented in Fig. (5 and Table 4).
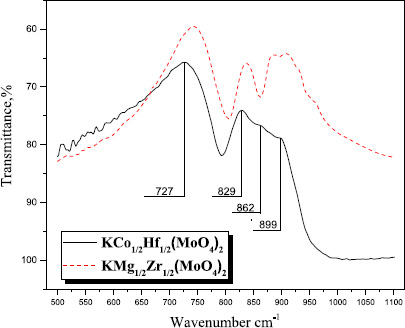
IR spectra of KCo1/2Hf1/2(MoO4)2 и KMg1/2Zr1/2(MoO4)2 [30].
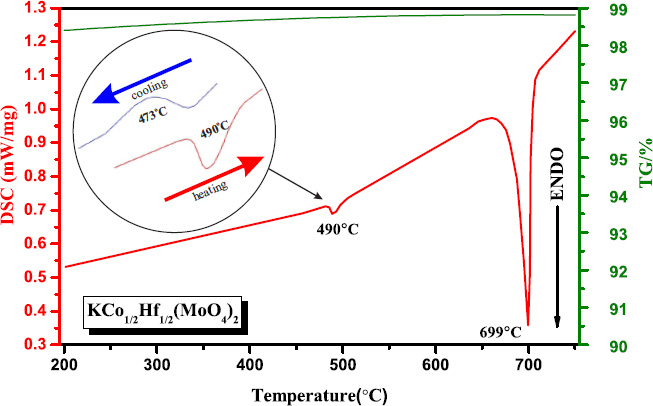
TG and DSC measurements of KCo1/2Hf1/2(MoO4)2.
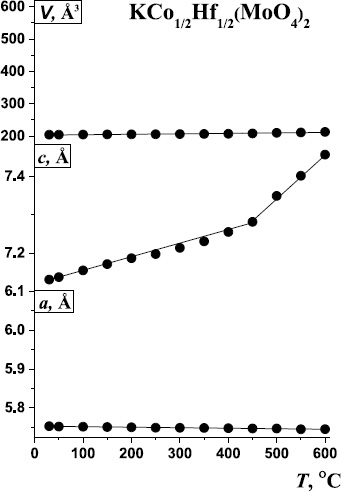
The dependence of the lattice parameters of KCo1/2Hf1/2(MoO4)2 on temperature.
T°C | a, Å | c, Å | V, Å3 |
---|---|---|---|
30.0 | 5.7528(7) | 7.131(1) | 204.38(6) |
50.0 | 5.7521(3) | 7.1374(7) | 204.51(3) |
100.0 | 5.7517(3) | 7.1550(7) | 204.99(3) |
150.0 | 5.7507(3) | 7.1716(7) | 205.39(3) |
200.0 | 5.7501(3) | 7.1869(7) | 205.79(3) |
250.0 | 5.7490(3) | 7.1977(7) | 206.02(3) |
300.0 | 5.7489(3) | 7.2140(7) | 206.48(3) |
350.0 | 5.7484(3) | 7.2308(8) | 206.92(3) |
400.0 | 5.7478(4) | 7.2553(8) | 207.58(4) |
450.0 | 5.7470(4) | 7.2817(8) | 208.28(3) |
500.0 | 5.7469(4) | 7.3494(7) | 210.21(3) |
550.0 | 5.7451(3) | 7.4021(6) | 211.58(3) |
600.0 | 5.7452(4) | 7.4574(7) | 213.17(3) |
The decrease in the a-parameter value is fairly linear over the entire temperature range, while the increase in the c-parameter can be described by two linear segments before and after 450°C. Thus, the point of curve inflection was observed on the temperature dependence curve of the c parameter around 450-500°С (which corresponds to the DSC data), followed by a more pronounced increase in this parameter. All reflections on high-temperature X-ray diffraction patterns in the entire studied temperature range are indicated in the same space group; no new peaks were detected, which indicates that the first-order phase transition is not accompanied by a change in the structure. The most likely cause of the phase transition is a change in the electronic structure of the Co2+ ion. The transition from the low-spin state to the high-spin state is accompanied by a sharp increase in the ionic radius from 0.79 to 0.885 A for the Co2+ ion with C.N. = 6 [31]. Such a transition was observed for the compound Co3BO5 [34]. The temperature-dependent variations of the lattice parameters were approximated using first-order polynomial functions (Table 5).
The coefficient of thermal expansion (CTE) calculated through the linear approximation of the temperature dependencies of the lattice parameters is shown in Table 6, with cross-sections of the CTE illustrated in Fig. (6). The thermal expansion of KCo1/2Hf1/2(MoO4)2 is notably anisotropic, with the greatest expansion observed along the c-axis.
The coefficient of thermal expansion (CTE) αc was 47×10–6 °С–1 up to 450°С and 156×10–6 °С–1, while αa remained negative at –2.3×10–6 °С–1 throughout the investigated temperature range. Despite this, no reduction in cell volume was observed. The values of αV for KCo1/2Hf1/2(MoO4)2 were 43×10–6 °С–1 at room temperature and 148×10–6 °С–1 at 600°С. These values are close to those calculated for the isostructural analogue KMg0.5Zr0.5(MoO4)2 (αV = 160×10–6 °С–1 at 500°С [30]).
The observed 2D negative thermal expansion in the ab plane is likely attributable to the thermal motion of the oxygen atom O2 (Wyckoff position 6i), as it is the only atom with the necessary degree of freedom. It is expected that, namely, the bond Co–O2 is the most “flexible”, that is, it has more freedom for a change. Thus, the unit cell expansion along the crystallographic с-axis can be attributed to an increase in the value of Co–O2 bond length.
3.3.1. Electrical Conductivity
The electrophysical properties of the examined ternary molybdate were also characterized. The temperature dependence of conductivity (in a heating-cooling cycle) at various frequencies and the impedance plot, which is typical for ionic conductors, are presented in Fig. (7).
Conductivity monotonically increased with rising temperature (100–450°C) from 10–9 to 10–6 S/cm. A subsequent sharp increase in conductivity was observed, reaching a value of 0.42·10–3 S/cm (570°C) with an activation energy Еа = 0.8 eV. A deviation from linearity in the temperature dependence of conductivity was observed during both heating and cooling, specifically at 510°C and 500°C, respectively. These values correlated with the temperature of the endothermic effect detected in the DSC curve (Fig. 4), indicating a first-order phase transition, which was further confirmed by the presence of thermal hysteresis (Fig. 7). The pattern of the temperature dependence of conductivity is similar to that of the isostructural molybdate KMg0.5Zr0.5(MoO4)2. Theoretical evaluation of the energy barriers of transport based on the bond valence sum maps (BVS) showed that its structure mainly realizes two-dimensional oxygen transport [30]. It is assumed that in KCo1/2Hf1/2(MoO4)2, the most probable charge carrier is also oxygen ions, which requires further research.
Table 5.
Cell | Т1, °С | Т2, °С | a0 | a1 | R2 |
---|---|---|---|---|---|
a | 30 | 600 | 5.7528(2) | -0.0130(5) | 1.000 |
c | 30 | 450 | 7.119(3) | 0.34(1) | 0.990 |
c | 450 | 600 | 6.78(3) | 1.14(5) | 0.996 |
V | 30 | 600 | 203.0(5) | 14(1) | 1.000 |
Т, °С | αa | αc | αV |
---|---|---|---|
30 | -2.26(9) | 47(2) | 43(7) |
50 | -2.26(9) | 47(2) | 43(7) |
100 | -2.26(9) | 47(2) | 43(7) |
150 | -2.26(9) | 47(2) | 43(7) |
200 | -2.26(9) | 47(2) | 42(7) |
250 | -2.26(9) | 47(2) | 42(7) |
300 | -2.26(9) | 47(2) | 42(7) |
350 | -2.26(9) | 47(2) | 42(7) |
400 | -2.26(9) | 47(2) | 42(7) |
450 | -2.26(9) | 46(2) | 42(7) |
500 | -2.26(9) | 155(7) | 151(7) |
550 | -2.26(9) | 154(7) | 150(7) |
600 | -2.27(9) | 153(6) | 148(7) |
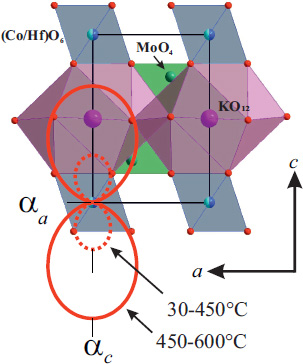
Projection of the KCo1/2Hf1/2(MoO4)2 structure on the ac plane, mapped to the thermal expansion tensor cross-section.
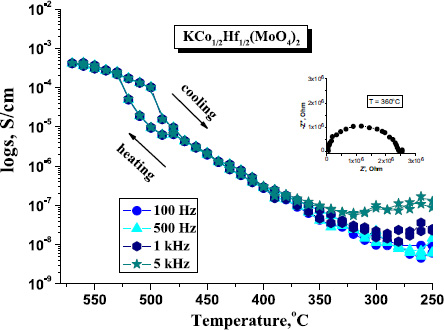
Temperature dependencies of electrical conductivity for KCo1/2Hf1/2(MoO4)2.
CONCLUSION
Ternary molybdate KCo1/2Hf1/2(MoO4)2 was synthesized via a ceramic route. Its structure was refined by the Rietveld method, with initial parameters based on literature data for the single crystal KMg0.5Zr0.5(MoO4)2 sample [32]. The compound studied was crystallized in the trigonal space group P3-m1. The experimental IR spectrum had characteristic lines of the MoO4 tetrahedra stretching modes, thus confirming the coordination of the Mo atoms in the refined structure. Differential scanning calorimetry (DSC) results demonstrated that KCo1/2Hf1/2(MoO4)2 melted at 699°C with decomposition. In this study, we investigated the ionic conductivity properties of KCo1/2Hf1/2(MoO4)2, which reached a conductivity value of 0.42·10–3 S/cm (570°C) with an activation energy Еа = 0.8 eV. Thermal deformations of KCo1/2Hf1/2(MoO4)2 were investigated using HT-XRD. The observed 2D negative thermal expansion in the ab plane did not lead to a reduction in the cell volume. Therefore, the studied ternary molybdate KCo1/2Hf1/2(MoO4)2 (αV = 148×10–6 °С–1 at 600°С) can be categorized as a high-expansion material [35].
AUTHORS’ CONTRIBUTIONS
It is hereby acknowledged that all authors have accepted responsibility for the manuscript's content and consented to its submission. They have meticulously reviewed all results and unanimously approved the final version of the manuscript.
LIST OF ABBREVIATIONS
NTE | = Negative Thermal Expansion |
HT-PXRD | = High-temperature Powder X-ray Diffraction |
CTE | = Coefficient of Thermal Expansion |
AVAILABILITY OF DATA AND MATERIALS
The data and supportive information are available within the article.
ACKNOWLEDGEMENTS
The authors would like to acknowledge the resources of the Research Equipment Sharing Center of BINM SB RAS (Ulan-Ude, Russia), which were used to carry out x-ray phase analysis, high-temperature x-ray diffraction, infrared spectroscopy, and conductivity measurements.